Catalysts speed up reactions by providing an alternative pathway that lowers the activation energy needed for the process. This makes it easier for molecules to collide with enough energy to react, increasing reaction rates without being consumed themselves. They work by forming temporary intermediates and activating reactants on surfaces or within solutions. If you’re curious how these tiny agents make reactions faster, you’ll find more interesting details below.
Key Takeaways
- Catalysts provide alternative pathways with lower activation energy, making reactions easier to proceed.
- They facilitate the formation of reaction intermediates, reducing the energy needed to reach the transition state.
- Catalysts enable reactants to collide with sufficient energy more efficiently, increasing reaction rates.
- Surface interactions and adsorption on catalyst surfaces activate molecules for faster bonding or breaking bonds.
- By regenerating after each reaction cycle, catalysts continuously accelerate reactions without being consumed.
Understanding Activation Energy and Reaction Rates
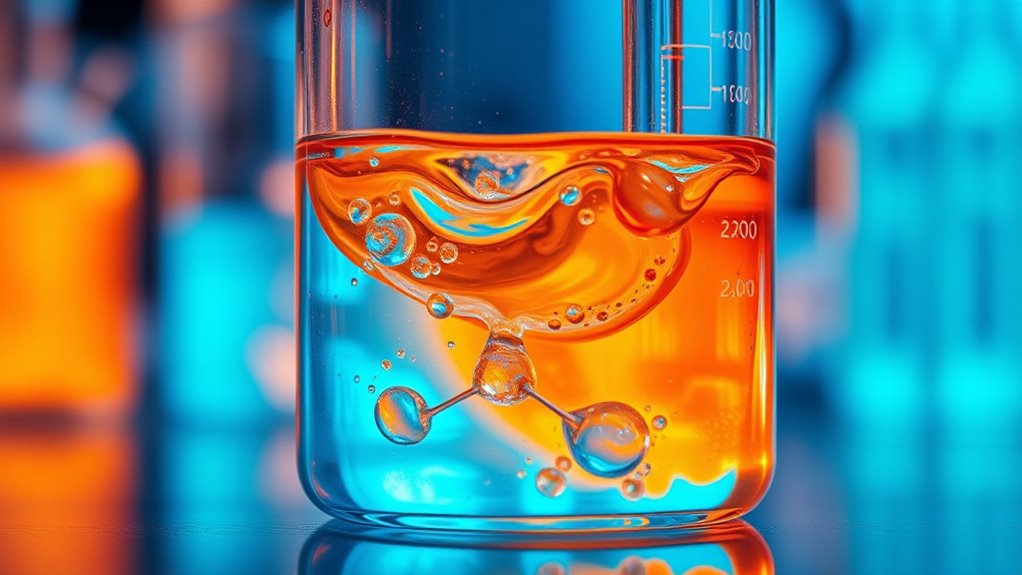
Have you ever wondered what determines how quickly a chemical reaction occurs? It all comes down to the energy barrier, known as activation energy ((E_a)), which reaction kinetics must overcome for bonds to break and new ones to form. The higher the energy barrier, the slower the reaction. This minimum energy threshold is measured experimentally using rate constants at different temperatures. According to the Arrhenius equation, even small decreases in (E_a) can considerably speed up reactions. Particles need to collide with enough energy to surpass this barrier, making temperature a critical factor. Activation energy represents the energy barrier that must be overcome for a reaction to proceed. Understanding the energy barrier helps you predict reaction rates and design catalysts that lower (E_a), thereby accelerating reactions efficiently.
The Role of Alternative Reaction Pathways
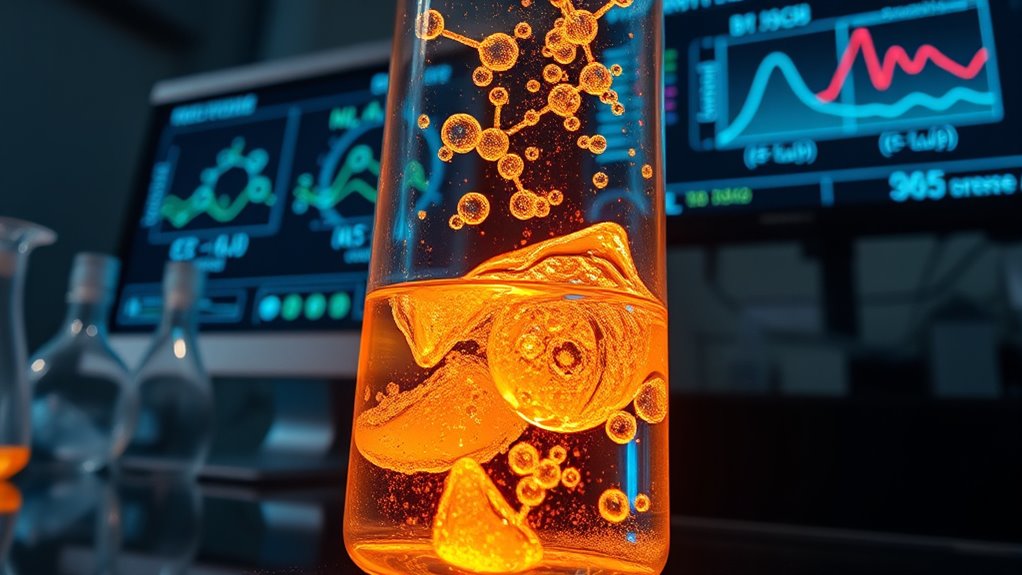
While lowering activation energy speeds up reactions, catalysts also work by providing entirely different reaction pathways. These alternative routes often involve reaction intermediates, which form temporarily during the process, making it easier for reactants to transform into products. Surface adsorption plays a key role here, especially in heterogeneous catalysis, where reactants attach to the catalyst’s surface. This process activates molecules, aligning them for more efficient bonding and breaking of bonds. By creating new pathways with lower energy barriers, catalysts enhance reactivity without changing the reactants or products. These alternative pathways allow reactions to proceed faster and more efficiently, as reactants are guided through specific intermediate stages on the catalyst’s surface, making the entire process smoother and more effective. Catalysts also provide an entirely different reaction pathway, which can significantly increase the overall reaction rate. Additionally, these pathways often involve reaction intermediates, which facilitate the transformation process. Incorporating reaction mechanisms into the study of catalysis offers deeper insights into how these processes accelerate chemical reactions. Furthermore, understanding the catalytic surface interactions is crucial for designing more effective catalysts for various industrial applications. For example, surface interactions can be tailored to optimize catalytic performance and selectivity.
How Catalysts Are Regenerated During Reactions
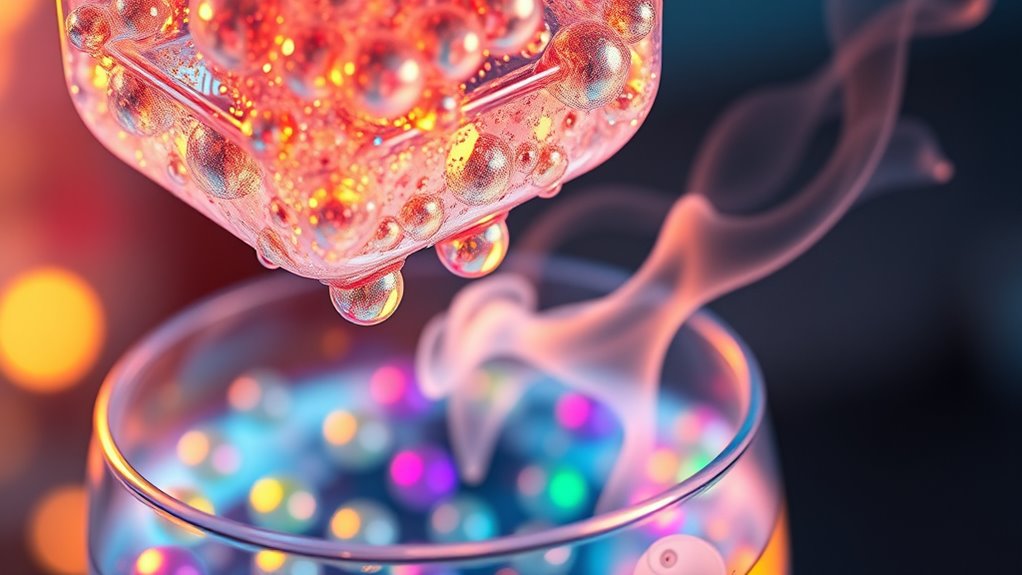
Catalysts become less effective over time because they accumulate deposits and contaminants that block active sites, shortening their catalyst lifespan. To restore their activity, industries use regeneration techniques like thermal treatment, which involves high temperatures to burn off deposits, carefully controlled to avoid damaging the catalyst. Chemical methods, such as acidic treatments, can also remove impurities while preserving active sites. Sometimes, a combination of thermal and chemical approaches is employed for more effective regeneration. This process is vital for maintaining efficiency and reducing costs in industrial applications. Proper regeneration techniques extend catalyst lifespan, minimize waste, and support sustainable operations. Advanced analytical techniques, including electron microscopy and porosity analysis, are essential for assessing catalyst condition and guiding regeneration processes. Additionally, monitoring methods help ensure that catalysts are regenerated correctly and maintain their activity over multiple cycles. Technological innovations are continuously improving regeneration methods, making them more efficient and environmentally friendly. By carefully managing regeneration, you guarantee catalysts remain active and effective over multiple reaction cycles, which is crucial for industrial sustainability. Moreover, understanding the catalyst deactivation mechanisms can help develop more targeted and effective regeneration strategies. Furthermore, implementing preventive maintenance strategies can reduce the frequency of regeneration needed, thereby saving costs and improving operational reliability.
Types of Catalysts and Their Unique Functions
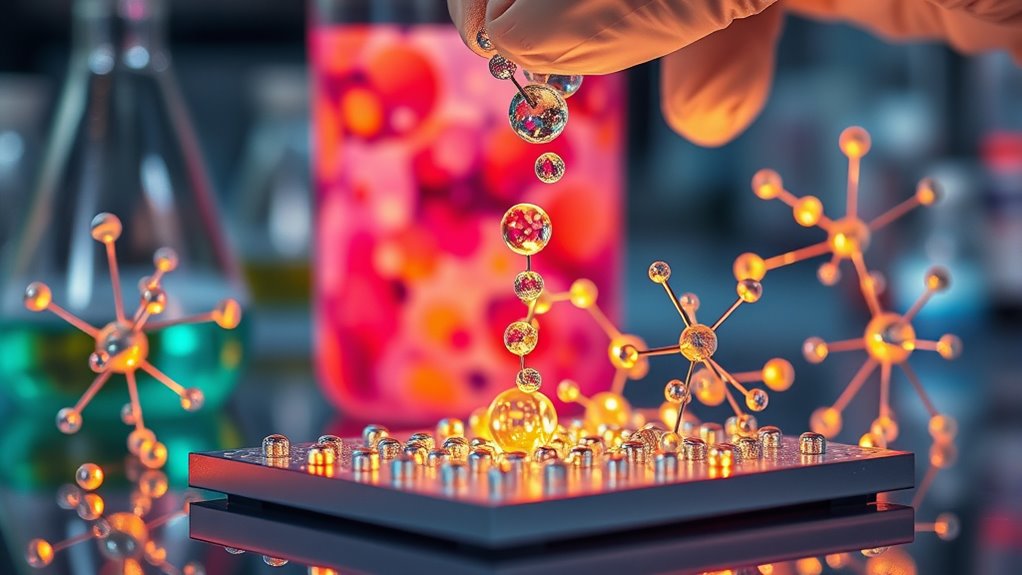
Different types of catalysts serve distinct roles in various reactions, each with unique functions that optimize efficiency. Enzymes, as biological catalysts, offer high catalyst selectivity, targeting specific substrates like lactose with precision. Enzymes are highly efficient and can operate under mild conditions, making them ideal for many biological and industrial processes. Acid-base catalysts donate or accept protons to speed reactions such as esterification. Heterogeneous catalysts, like iron in ammonia synthesis, operate on surface sites and are easily separated, but they can suffer from catalyst deactivation if surface sites foul or become blocked. Surface fouling can significantly reduce the activity of heterogeneous catalysts over time, requiring regeneration or replacement. Homogeneous catalysts, such as transition metal complexes, work in solution, providing uniform activity. Promoters and inhibitors further influence catalyst effectiveness. Recognizing these different types helps prevent catalyst deactivation and guarantees reactions proceed efficiently, leveraging each catalyst’s unique function to speed up processes without unnecessary side reactions. Additionally, using specialized catalyst designs can further enhance reaction rates and selectivity in specific applications. Moreover, understanding the energetic alignment of catalysts with reactants can significantly improve reaction efficiency and selectivity. Incorporating biocatalyst engineering techniques can further optimize biological catalysts for industrial use.
The Mechanisms Behind Catalyzed Reactions
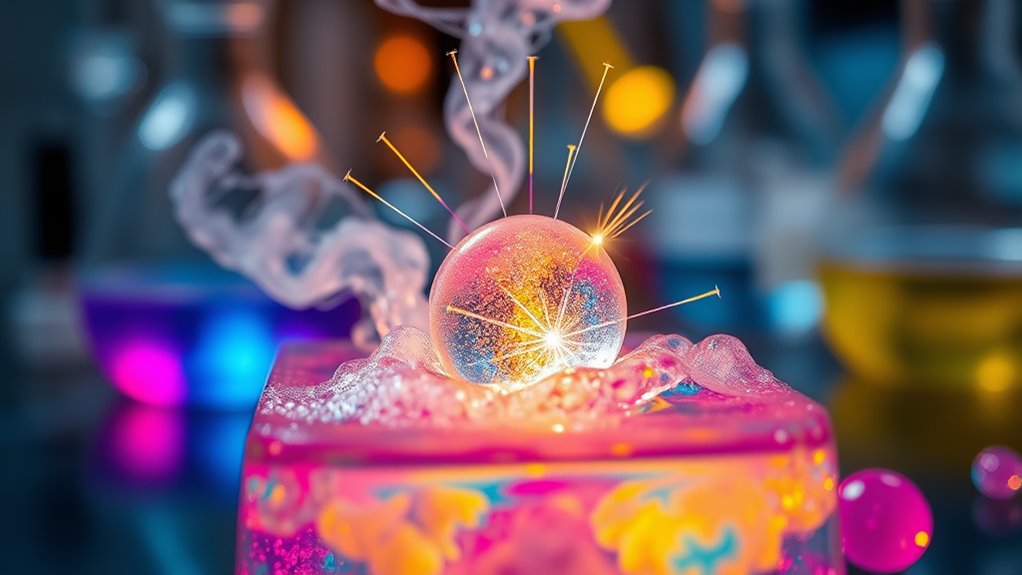
Understanding how catalyzed reactions work requires examining the mechanisms that enable them to proceed more efficiently. Catalyst surface interactions play a key role, as they allow reactants to bind to the catalyst, forming intermediates that lower the activation energy needed for the reaction. Enzyme specificity ensures that only particular reactants fit into active sites, facilitating precise interactions. These interactions create changeover states with reduced energy barriers, speeding up the process. The reaction often involves multiple steps, with intermediates forming and being consumed, allowing the catalyst to regenerate and repeat the cycle. Each step’s shift state is critical, helping to accelerate the overall reaction. Understanding catalyst mechanisms provides insight into how catalysts notably enhance reaction rates by providing alternative pathways with lower activation energy. Additionally, the reaction pathway can be significantly altered through various catalytic processes, further increasing efficiency. Incorporating catalyst surface interactions enhances our comprehension of how the physical contact between catalyst and reactants influences the reaction speed.
Practical Uses of Catalysts in Industry and Nature
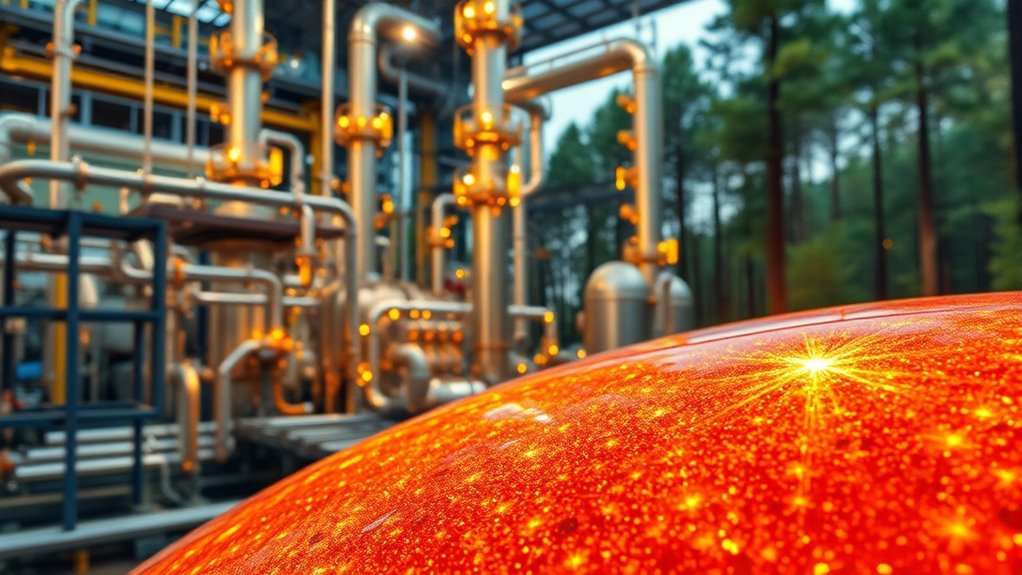
Many industries rely on catalysts to improve efficiency and produce desired outcomes. In agriculture, catalysts help synthesize fertilizers like ammonia through the Haber-Bosch process, boosting crop yields. Catalysts in the textile industry accelerate chemical reactions to produce dyes and fibers more efficiently, reducing waste and energy use. These catalysts enable cleaner manufacturing processes and higher-quality products. In addition, catalysts in pollution control, such as catalytic converters, reduce vehicle emissions by converting harmful gases into less harmful substances. Natural processes also benefit from catalysts, with enzymes speeding up essential biological reactions. Catalysts are also used in the production of plastics and synthetic materials. Understanding reaction mechanisms can help optimize catalyst performance and develop new catalytic processes. Overall, catalysts play a pivotal role in making industrial processes more sustainable and effective, supporting both economic growth and environmental protection.
Benefits and Challenges of Using Catalysts
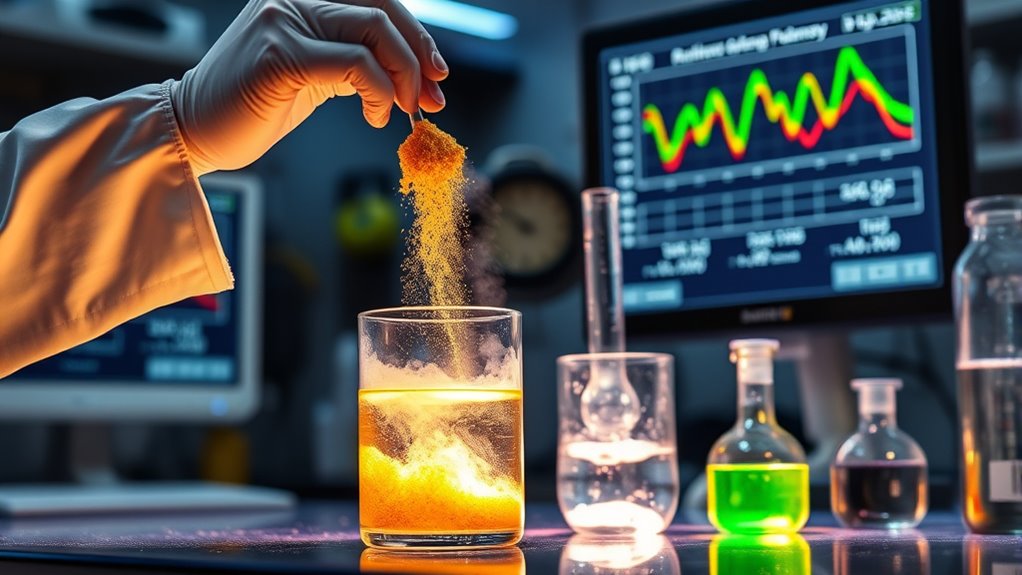
Using catalysts offers significant advantages in chemical processes, primarily by speeding up reactions and making them more energy-efficient. Catalyst discovery plays a pivotal role in improving efficiency and selectivity, which can lead to faster market adoption. New advancements in catalyst materials are continually emerging to enhance performance and sustainability. Furthermore, ongoing research into AI security helps identify potential vulnerabilities in catalyst development processes, ensuring safer implementation. However, there are challenges too. Developing effective catalysts is costly, often relying on rare materials, and their stability can degrade over time, requiring replacements. Designing catalysts that balance activation energy reduction with high selectivity is complex. Additionally, scaling production while maintaining quality remains a hurdle. Despite these challenges, the benefits—such as increased productivity, reduced energy costs, and environmental gains—make catalysts indispensable. As research advances, new materials and technologies promise to address existing issues, boosting market adoption and expanding their benefits across industries. Catalysts lower the activation energy needed for reactions, which further enhances their efficiency and effectiveness.
Frequently Asked Questions
Can Catalysts Work in All Types of Chemical Reactions?
You might wonder if catalysts work in all types of reactions. While they can speed up many reactions by altering reaction mechanisms, they don’t work in every case. Catalysts are selective; they only accelerate specific pathways and can’t overcome thermodynamic barriers or drive non-spontaneous reactions. Factors like reaction type, phase, and catalyst stability influence their effectiveness. So, not all reactions benefit from or even allow catalysts to operate efficiently.
How Do Biological Enzymes Differ From Industrial Catalysts?
Imagine you’re choosing a tool for a delicate task—you’ll want the right fit. Biological enzymes differ from industrial catalysts because of their enzyme specificity, acting on specific substrates and being made of proteins. Industrial catalyst types, on the other hand, are more versatile but less selective. Enzymes operate under mild conditions, making them eco-friendly, while industrial catalysts often require harsher environments for their broader applications.
What Environmental Impacts Do Catalysts Have?
You might wonder about catalysts‘ environmental impacts. Catalysts, especially sustainable ones like enzymes and SACs, help reduce environmental pollution by lowering emissions, hazardous waste, and toxic intermediates. They also promote resource conservation by increasing yields, decreasing raw material use, and enabling milder process conditions. Using eco-friendly catalysts minimizes energy consumption and waste, making industrial processes cleaner and more sustainable for the environment and future resource availability.
Are Catalysts Always Cost-Effective for Industrial Use?
Imagine you’re in a bustling factory, where catalysts are the unsung heroes. Are they always cost-effective? Not necessarily. You need to weigh the economic benefits against costs, considering catalyst lifespan and efficiency. Investing in durable, high-performance catalysts can boost productivity and reduce expenses over time. However, initial costs or frequent replacements might outweigh benefits. Carefully analyze your process, optimize catalyst use, and you’ll find the balance for cost-effective, sustainable operations.
How Do Temperature and Pressure Influence Catalyst Efficiency?
You see, temperature and pressure directly impact catalyst efficiency by influencing reaction mechanisms and activation energy. Higher temperatures increase molecular collisions, lowering activation energy and speeding up reactions. Elevated pressure boosts reactant concentration, enhancing contact with catalyst surfaces. Together, these conditions optimize reaction pathways, making catalysts work more effectively. But, too much heat or pressure can deactivate catalysts or cause structural issues, so finding the right balance is key.
Conclusion
Now that you understand how catalysts lower activation energy and create alternative pathways, you can see their power in speeding up reactions. They make processes more efficient without being consumed themselves. Imagine the impact if we applied this knowledge broadly—could we solve some of today’s biggest challenges? Catalysts aren’t just tools; they’re catalysts for change, inspiring innovation and sustainability. So, aren’t you curious how embracing catalysts could transform our world for the better?