Ice floats on water because it’s less dense due to its unique molecular structure and hydrogen bonding. When water freezes, hydrogen bonds arrange molecules into an open, spacious hexagonal lattice that creates empty spaces. This structure causes ice to expand and become less dense than liquid water, allowing it to float. To discover how this fascinating process works and why it’s so important, continue exploring the detailed science behind ice’s buoyancy.
Key Takeaways
- Ice has a lower density (~0.917 g/cm³) than liquid water, causing it to float due to buoyancy principles.
- Hydrogen bonding in ice creates a crystalline, open hexagonal lattice that increases its volume and decreases its density.
- The open lattice structure contains cavities and voids, making ice less dense than water.
- The molecular arrangement in ice keeps molecules farther apart, reducing overall density compared to liquid water.
- Because of its lower density, ice displaces a weight of water equal to its own, allowing it to stay afloat.
The Unique Density of Ice Compared to Water
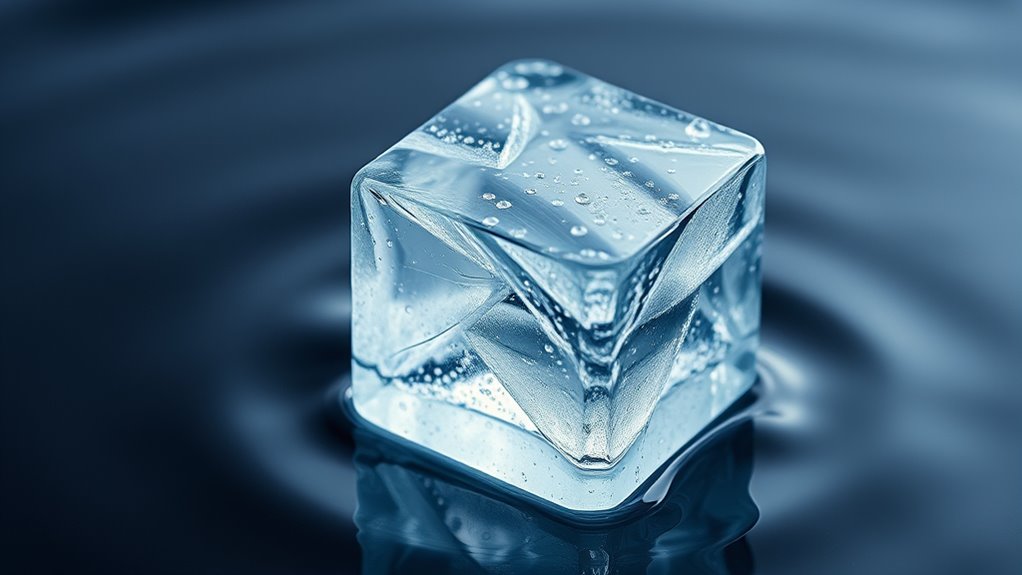
Ice has a surprisingly low density compared to liquid water, which is why it floats. This phenomenon stems from water’s unique chemical properties, especially hydrogen bonding, which creates an open, rigid lattice in ice. Because of this structure, ice occupies about 9% more volume than the same amount of water, reducing its density to roughly 0.917 g/cm³. Additionally, ice’s thermal conductivity is lower than that of liquid water, meaning it’s less efficient at transferring heat. This helps ice insulate the water below, maintaining a stable environment for aquatic life. The low density isn’t just a quirk; it’s a direct result of water’s molecular arrangement and thermal properties, making ice a remarkable exception among solids. The structure of ice is responsible for its low density. Furthermore, the hydrogen bonds in water are responsible for creating the open lattice structure in ice, which is less dense than liquid water. This unique arrangement also influences water’s melting point, which is relatively high compared to other substances of similar molecular weight, further emphasizing water’s unusual properties.
How Molecular Structure Creates Space in Ice
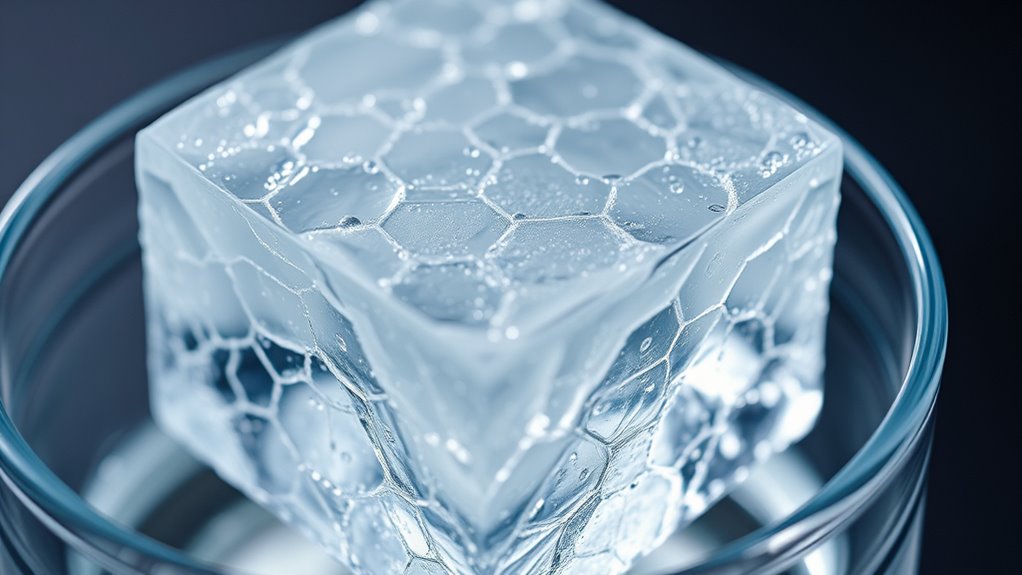
The molecular arrangement in ice forms a hexagonal crystalline lattice that inherently creates open spaces between water molecules. This structure results from molecular symmetry, where water molecules align to maximize hydrogen bond distances, forming a regular hexagonal pattern. The crystalline lattice’s geometry prevents tight packing, leaving voids or empty spaces within the structure. These open channels are a direct consequence of the lattice’s symmetry and geometric constraints, which resist efficient molecular packing. As a result, about 10% of ice’s volume consists of these voids, creating a less dense solid than liquid water. This crystalline lattice’s unique arrangement explains why ice is less dense and floats, maintaining the open spaces that are a hallmark of its molecular structure. Additionally, the hexagonal shape of ice’s crystal structure contributes to its ability to form a stable, open framework. The open, porous nature of this structure also influences thermal insulation, making ice a good insulator due to the trapped air in its lattice.
The Role of Hydrogen Bonding in Ice Formation
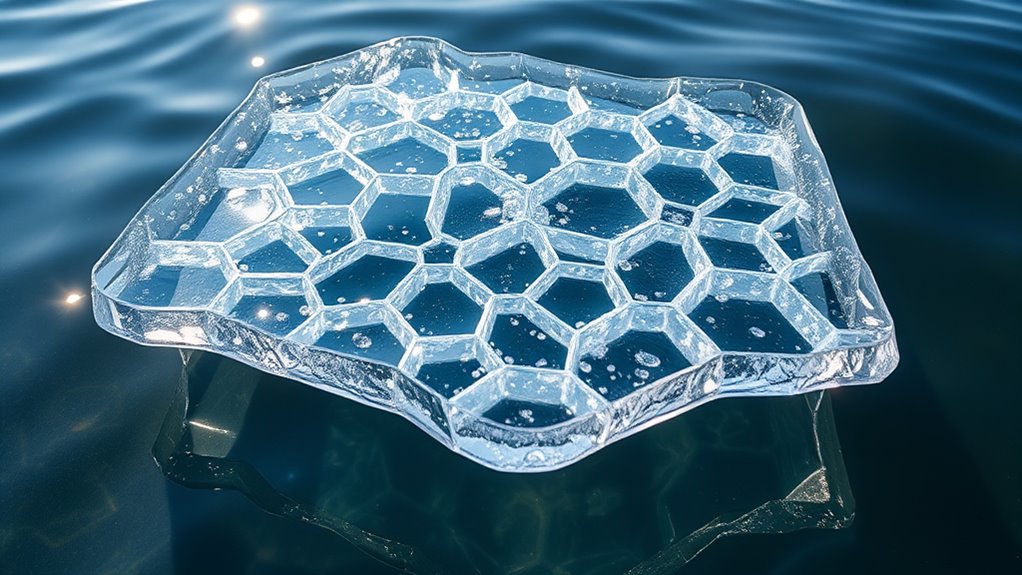
Hydrogen bonding is fundamental to how water molecules organize themselves into the rigid, crystalline structure of ice. These hydrogen bonds form between the partial positive charge of hydrogen atoms and the partial negative charge on oxygen in neighboring molecules. This intermolecular attraction guides a specific molecular arrangement, where each oxygen atom links with four hydrogens in a tetrahedral shape. The directional nature of hydrogen bonds creates a stable, hexagonal lattice with open channels, resulting in a low-density, low-volume structure. This ordered bonding pattern guarantees that ice maintains a rigid, crystalline form, differentiating it from liquid water’s more disorganized, transient bonds. Additionally, the molecular structure of ice is responsible for its lower density compared to water. The geometric arrangement of molecules caused by hydrogen bonds is crucial in understanding why ice floats. The strength and directionality of hydrogen bonds are key to shaping ice’s unique molecular arrangement and physical properties. Furthermore, this specific bonding pattern leads to the unique physical property of ice floating, as the open lattice structure makes it less dense than liquid water. To better understand this phenomenon, it is helpful to consider the temperature dependence of hydrogen bonding and how it influences phase changes in water.
Volume Expansion During Freezing
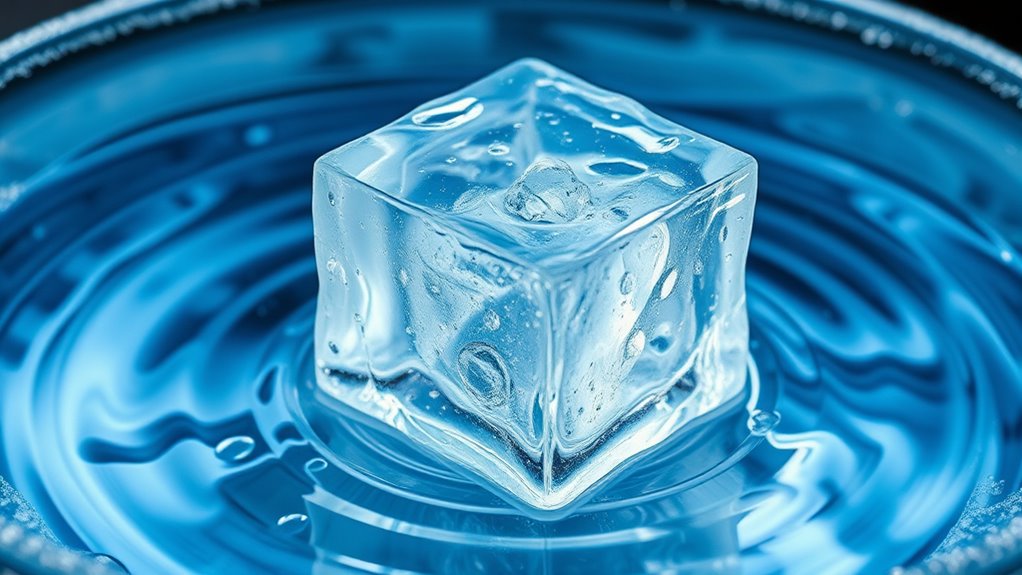
When water freezes, it expands by about 9% in volume, a phenomenon that surprises many because most substances contract upon solidification. This volume expansion results from water’s unique thermal properties during phase transitions. As temperature drops below 4°C, the hydrogen bonds rearrange into a hexagonal lattice, creating open spaces that increase volume. This crystalline structure, with its cage-like arrangement, leaves roughly 10% empty space compared to liquid water. The expansion is influenced by pressure; higher pressure inhibits freezing and reduces expansion. This phenomenon has real-world impacts, such as causing pipe bursts and rock weathering. Water’s unique molecular structure is key to understanding why ice floats and expands when it solidifies. Additionally, the properties of hydrogen bonding are fundamental to this anomalous behavior, as they determine the arrangement and stability of water molecules during freezing. Understanding this volume change is crucial for grasping water’s anomalous behavior and how phase transitions influence its thermal properties, especially during freezing.
Principles of Buoyancy and Archimedes’ Law
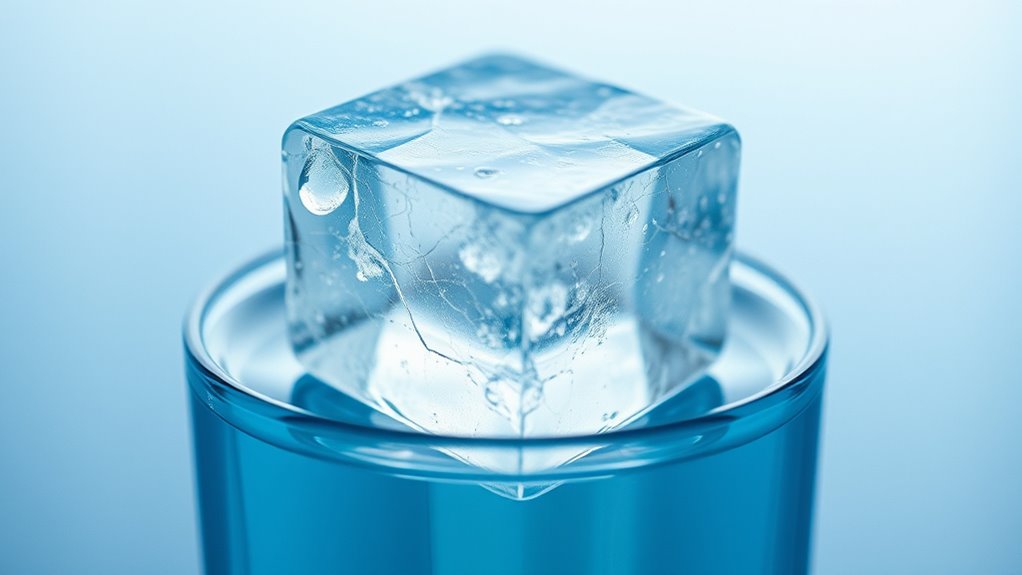
Have you ever wondered why objects float or sink in water? It all comes down to the principles of buoyancy and Archimedes’ law. Buoyancy is the upward force a fluid exerts on an immersed object, caused by pressure differences. When you consider an object’s density relative to water, it determines whether it floats or sinks. Archimedes’ law states that the buoyant force equals the weight of displaced fluid, calculated as F(_b = rho V g). While thermal conductivity influences how heat moves through materials, the melting point impacts the state of water and ice, affecting buoyancy. Understanding the role of density helps clarify why some objects float while others sink. Additionally, the density of water varies with temperature and impurities, which further influences buoyancy effects. A comprehensive understanding of fluid properties enhances our grasp of why ice, with its unique melting point and low density, floats, illustrating the balance of forces at play in fluid mechanics. Moreover, pressure differences within the fluid also play a crucial role in the overall behavior of floating objects. The buoyant force is present regardless of whether the object floats or sinks, illustrating the balance of forces at play in fluid mechanics. Recognizing how density and buoyancy interact can help in understanding various natural and engineering phenomena. Understanding these principles explains why ice, with its unique melting point and low density, floats, illustrating the balance of forces at play in fluid mechanics.
The Impact of Density on Ice’s Ability to Float
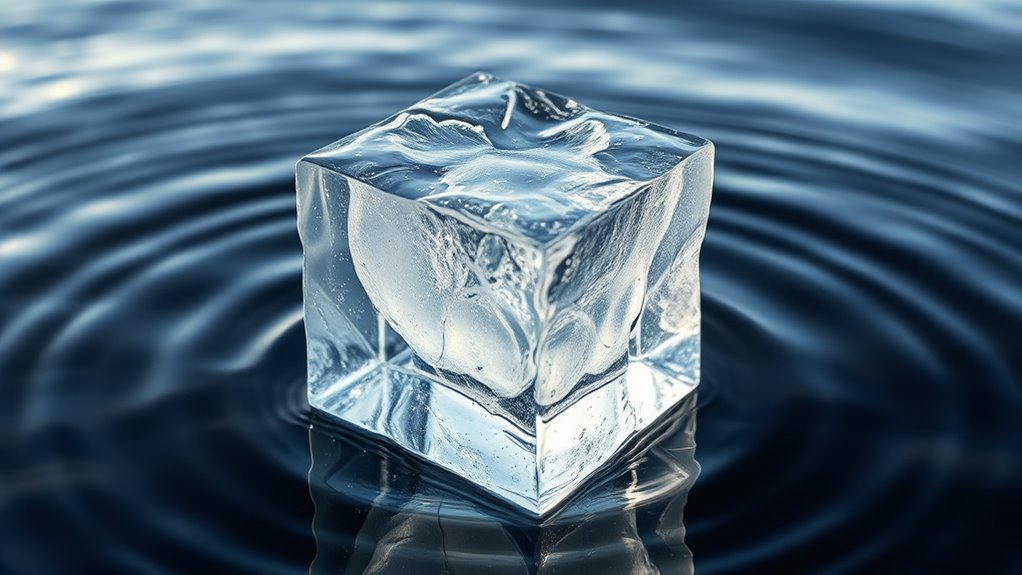
The ability of ice to float on water hinges on its density, which is markedly lower than that of liquid water due to its molecular structure. Ice forms a rigid tetrahedral arrangement, creating cage-like spaces that reduce its density. Its chemical properties, especially hydrogen bonding, hold molecules farther apart, making ice about 9% less dense than water. This lower density causes ice to float, unlike most solids. Additionally, ice’s thermal conductivity is lower than that of liquid water, allowing it to act as an insulator. This insulation helps preserve liquid water underneath, supporting aquatic life. The crystalline structure of ice, combined with its molecular arrangement, directly influences its buoyancy, illustrating how density and chemical properties determine ice’s ability to float. Moreover, density differences are crucial in natural processes such as glacial movement and climate regulation. Understanding biodiversity in aquatic ecosystems highlights the importance of ice’s insulating properties in maintaining healthy habitats for diverse species. The thermal properties of ice also play a vital role in controlling seasonal changes in climate and weather patterns. Furthermore, the unique phase transition properties of ice are essential in various geological and environmental processes. Recognizing the molecular arrangement of ice helps explain its physical behavior and its role in Earth’s climate systems.
Environmental Significance of Floating Ice
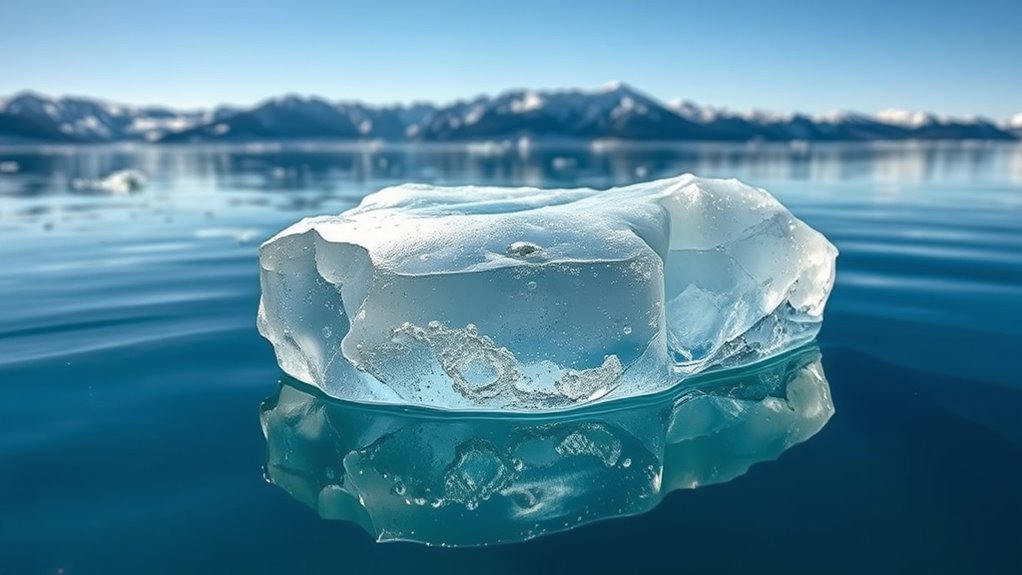
Floating ice plays a vital role in shaping Earth’s environment by providing insulation and water protection in cold regions. It maintains aquatic ecosystems during winter by insulating water below and reducing evaporative losses, which helps preserve water quality and stabilizes temperatures. [The ecological importance of floating ice extends to supporting habitats for algae, krill, seals, and polar bears, ensuring biodiversity and ecosystem stability.] Climate implications are significant; ice loss disrupts thermohaline circulation, accelerates regional warming through the albedo effect, and affects precipitation patterns. Research indicates that the presence of ice also influences ocean currents, which in turn regulate global climate systems. Indigenous communities rely on stable ice for hunting and transportation, while declining ice impacts tourism and fisheries. Overall, floating ice acts as a buffer against climate change, regulating environmental processes critical to maintaining Earth’s delicate balance. The high energy requirement for changing states also helps regulate temperature fluctuations and maintain environmental stability. Additionally, the thermal properties of ice contribute to its ability to modulate climate by absorbing and releasing heat slowly, which further stabilizes regional temperatures. Furthermore, the insulating capacity of ice is essential in protecting underlying ecosystems from extreme cold and temperature swings, reinforcing its environmental significance.
Demonstrating Density Differences Through Experiments
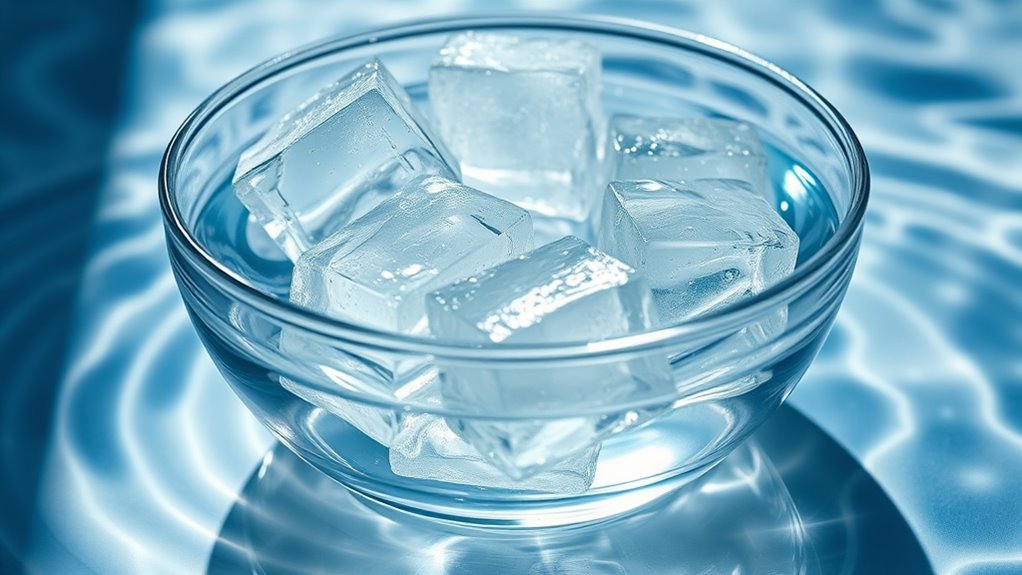
Demonstrating density differences through experiments offers a clear and engaging way to understand how objects and liquids behave based on their mass and volume. You can use simple setups to visualize these differences through experimental visualization, such as layering liquids with varying densities. By measuring density—calculated as mass divided by volume—you see how substances like ice, water, and oils separate or float. For example, adding food coloring enhances visibility, making layers more distinct. Temperature control and careful pouring prevent mixing, allowing you to observe how denser liquids settle below less dense ones. These experiments help grasp core concepts of density measurement and physical properties, illustrating why ice floats on water and how different materials interact based on their density. Understanding these principles is fundamental to grasping many natural phenomena involving buoyancy and material interactions. Conducting density experiments can also demonstrate how temperature and pressure influence material properties, providing deeper insights into the behavior of substances under various conditions. Incorporating AI-driven data analysis can further improve the accuracy and insights gained from such experiments.
Scientific Insights Into Ice’S Less Dense Structure
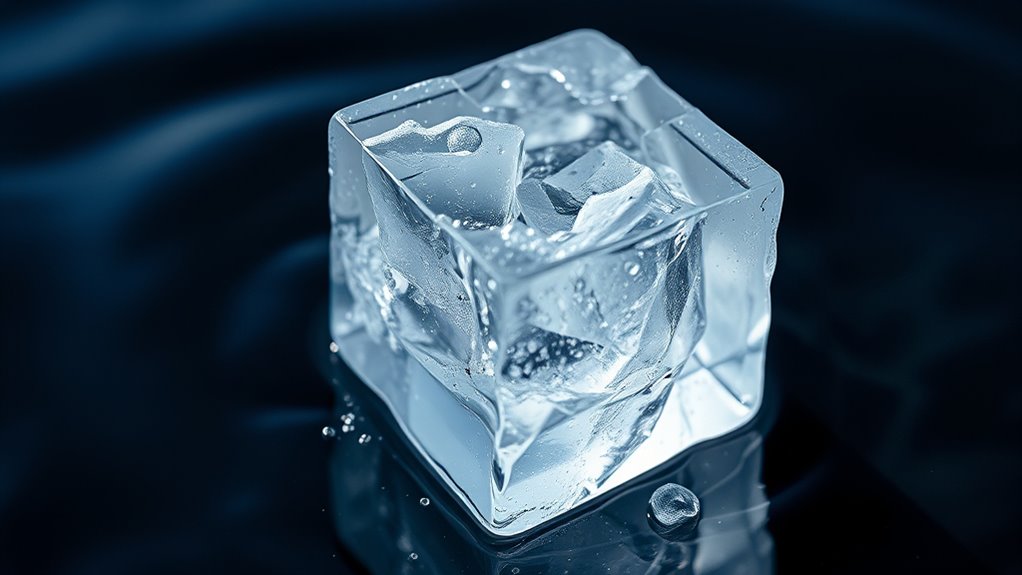
Understanding why ice floats requires examining its unique molecular structure. During the phase shift from water to ice, hydrogen bonds form a rigid, hexagonal lattice with empty spaces between molecules, creating a crystalline arrangement. This structure causes ice to occupy about 9% more volume than liquid water at 4°C, resulting in lower density. The bond angles—around 109.5°—prevent molecules from packing densely, unlike in liquid water where bonds break and reform continuously. Ice’s crystalline lattice contains cavities that reduce its mass per unit volume. Additionally, its thermal conductivity is lower than that of liquid water, allowing it to insulate and maintain its structure. These molecular and thermodynamic factors make ice less dense, enabling it to float on water. This molecular arrangement is a key factor in understanding the physical properties of ice.
Frequently Asked Questions
Why Is Ice Less Dense Than Water Despite Being a Solid?
You might wonder why ice is less dense than water. It’s because of its molecular arrangement: in ice, hydrogen bonds form a rigid, open tetrahedral lattice with gaps, leading to a density anomaly. This structure causes ice to expand upon freezing, making it less dense than liquid water. So, even as a solid, ice remains lighter and floats, thanks to its unique molecular organization and the resulting density difference.
How Does the Tetrahedral Structure of Ice Affect Its Density?
The tetrahedral structure transforms your water into a spacious, stable crystal lattice. This molecular arrangement, with angles of 109.5°, creates open hexagonal rings and void spaces, reducing density. Because rigid hydrogen bonds prevent molecules from packing tightly, ice’s crystal lattice is less compact than liquid water. This spacious structure causes ice to be lighter, enabling it to float, as the open molecular arrangement minimizes overall density.
What Role Do Hydrogen Bonds Play in Ice’s Formation and Buoyancy?
Hydrogen bonding is vital in forming ice’s crystalline structure, creating an open, hexagonal network that makes ice less dense. As you observe, these hydrogen bonds hold water molecules in a rigid, spacious arrangement. This structure results in lower mass per volume, causing ice to float. Basically, hydrogen bonds maintain the open framework, giving ice its buoyancy by making it less dense than liquid water.
Why Does Water Expand When It Freezes Into Ice?
Imagine water’s molecular dance during its phase shift, where it takes a new form. When water freezes, its molecules arrange into a crystalline lattice, creating more space between them. This molecular arrangement causes the volume to expand, making ice less dense. So, as water transitions from liquid to solid, it’s like a dance move that spreads out, causing the expansion that makes ice float.
How Does Ice’s Lower Density Influence Aquatic Ecosystems?
You might not realize it, but ice’s lower density plays a crucial role in aquatic ecosystems. It creates an insulating layer that helps keep water beneath from freezing completely, which is essential for aquatic life survival. This floating ice preserves habitats like polar bear environments, allowing them to hunt and breed. It also maintains oxygen levels and temperature stability, ensuring the health of underwater communities during winter.
Conclusion
Ever wonder why ice floats on water? It all comes down to its unique molecular structure and hydrogen bonding, which make ice less dense than liquid water. This fascinating property isn’t just a scientific curiosity — it plays an essential role in Earth’s environment. So next time you see ice floating, ask yourself: isn’t it amazing how such tiny molecules create such a big impact? Understanding this helps us appreciate nature’s delicate balance even more.