Transition metals are elements with partially filled d orbitals, giving them unique properties. They can exist in multiple oxidation states, forming colorful and complex compounds. These metals have high melting points, good conductivity, and are often magnetic. Their electrons enable strong bonding and diverse reactions. If you’re curious about how these characteristics influence their uses and behaviors, there’s much more to explore below.
Key Takeaways
- Transition metals have partially filled d subshells, enabling unique chemical and physical properties.
- They can exhibit multiple oxidation states, facilitating diverse redox reactions.
- Their valence electrons occupy both s and d orbitals, affecting bonding and reactivity.
- Transition metals are often colorful due to d-d electron transitions.
- They are known for high conductivity, density, and magnetic properties.
Defining Characteristics of Transition Metals
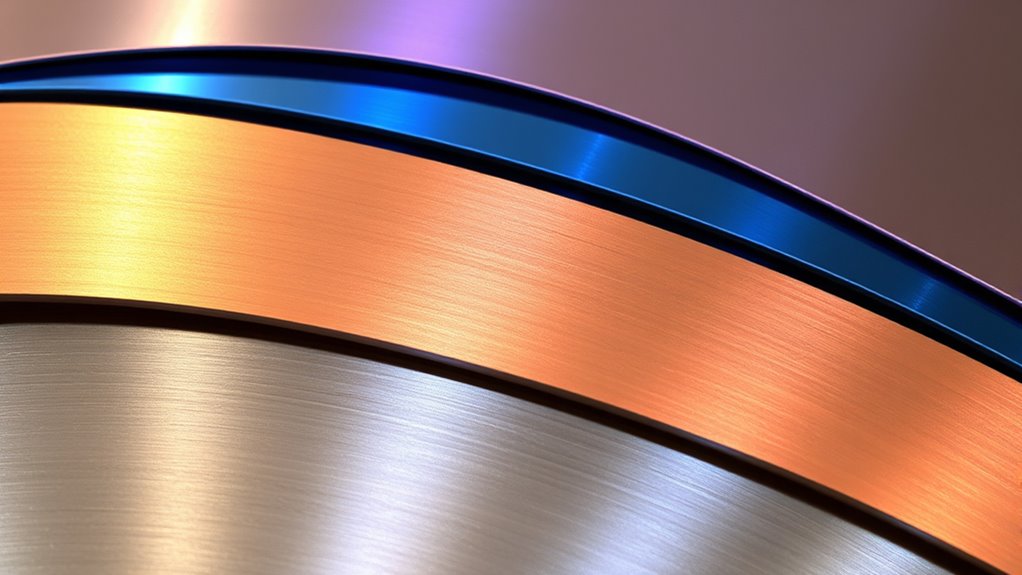
Transition metals are characterized by their unique electronic configurations, which include partially filled d subshells in atomic or ionic states. This means that in their neutral or charged forms, their d orbitals aren’t fully filled, giving these metals distinctive properties. Their partially filled d orbitals allow for diverse chemical behaviors. Your valence electrons occupy both s and d orbitals, enabling complex interactions. Thanks to d-d electron shifts, you can observe their striking optical properties, like vibrant colors in solutions. Electron delocalization within metallic bonds makes them good conductors of heat and electricity. Their variable oxidation states stem from the accessibility of d-electron electrons, allowing them to switch between different charges easily. These variable oxidation states enable transition metals to participate in a wide range of redox reactions, which is crucial for their industrial applications. Additionally, the d-electron configurations contribute to their catalytic abilities in various industrial processes. Their electronic structure also facilitates complex formation, allowing transition metals to form stable coordination compounds with a variety of ligands.
Physical and Chemical Properties
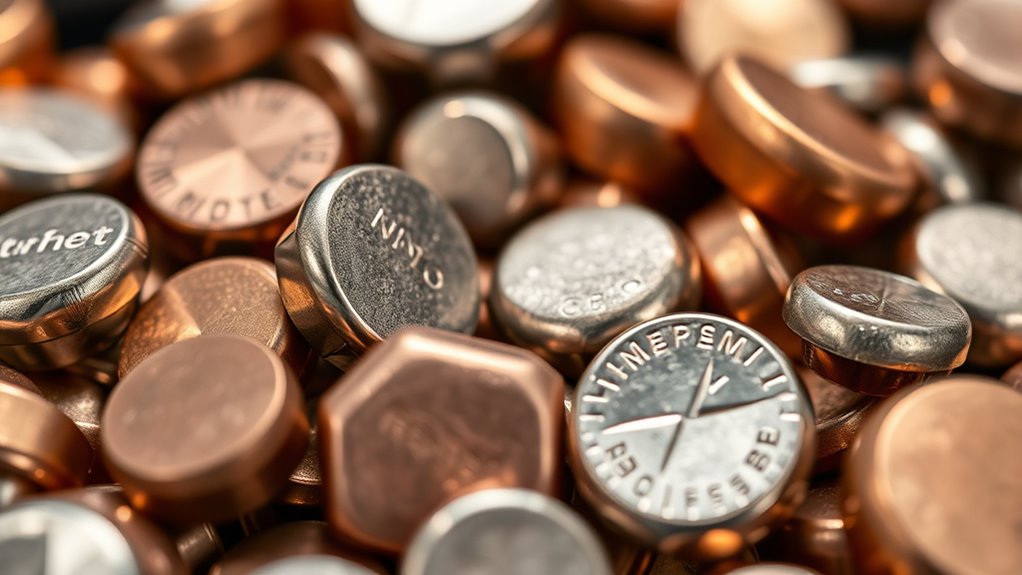
The unique electronic configurations of transition metals influence their physical and chemical behaviors. You’ll notice they’ve high melting and boiling points because delocalized d electrons strengthen metallic bonds. Their atoms are dense and hard, thanks to a compact atomic structure, and they form metallic lattice structures that support electron delocalization. Additionally, their ability to form multiple oxidation states is linked to their electron configurations, which vary across the period and group. These metals conduct electricity well because of numerous free electrons, with copper being especially notable for wiring. Physical properties vary across the periodic table, affected by nuclear charge and electron arrangements. Their variable oxidation states allow them to participate in a wide range of chemical reactions, enhancing their versatility. Chemically, they show multiple oxidation states and form colorful compounds due to d-d electron transitions. Many are paramagnetic because of unpaired d electrons. They also act as catalysts, form stable complexes, and engage in redox reactions, making transition metals highly versatile.
Electron Configuration and Bonding Behavior
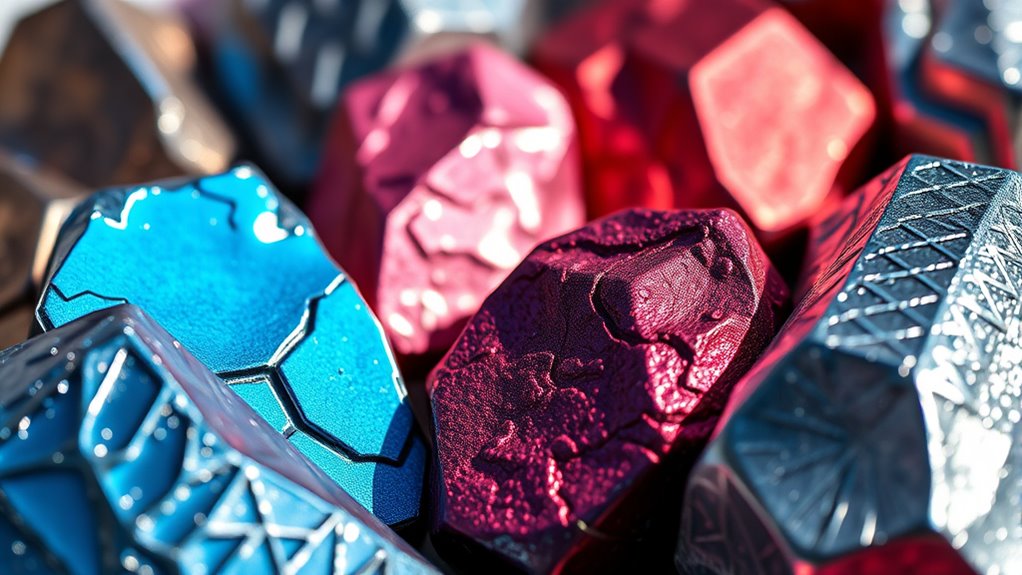
How do the electron configurations of transition metals influence their bonding behavior? Their configurations start with an argon core plus 4s²3dⁿ electrons, which are key to bonding. The 4s electrons fill first but are removed before 3d electrons during ionization, allowing multiple oxidation states. Unpaired d-electrons enable paramagnetism and redox versatility, while their involvement in covalent bonds creates directional coordination complexes. Hybridization (e.g., sp³d²) forms stable geometries like octahedral shapes. D-orbitals also participate in π-backbonding, strengthening metal-ligand bonds. Delocalized d-electrons contribute to metallic bonding, giving transition metals high electrical conductivity and thermal stability. Their versatile electron configurations and bonding modes, including ligand interactions and charge transfers, underpin their diverse chemical reactivity and catalytic properties. Additionally, metal-ligand bonding plays a crucial role in their catalytic functions, leveraging the flexible electronic structures of transition metals. The ability of transition metals to adopt various oxidation states results in variable oxidation states, which is essential for their catalytic activity in many industrial processes.
Industrial Uses and Applications
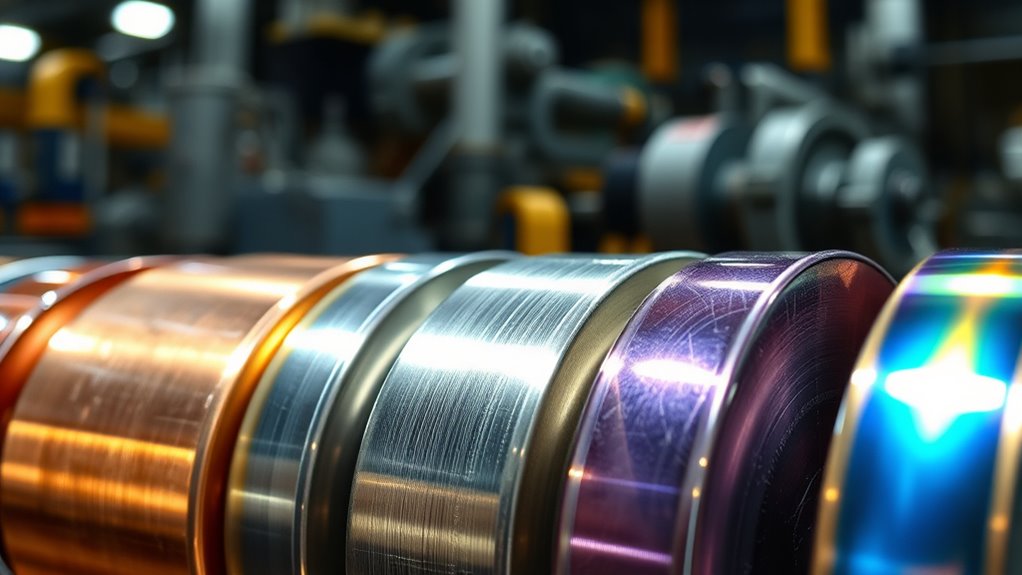
Transition metals’ versatile electron configurations enable a wide range of industrial applications. Cobalt is essential in lithium-ion batteries, powering electric vehicles and gadgets. Nickel’s role in NiMH batteries and emerging solid-state options makes it necessary for energy storage. Rare earth metals like scandium and yttrium are also used to enhance material properties, although their rarity limits widespread deployment. Additionally, their variable oxidation states allow them to participate in diverse chemical reactions, increasing their industrial value. Titanium’s strength and corrosion resistance make it ideal for wind turbine components. Copper’s excellent electrical conductivity is critical in solar panel wiring and power transmission. Vanadium is being explored for vanadium redox batteries, offering promising large-scale energy storage solutions. Advanced energy storage solutions increasingly rely on vanadium-based technologies to support renewable integration. In catalysis, platinum, palladium, and rhodium nanoparticles facilitate hydrogenation and reduce environmental impact. Nickel and vanadium compounds serve in industrial reactions, enhancing efficiency. These metals form durable alloys used in machinery, turbines, and medical devices. Their unique properties drive innovations across energy, manufacturing, and environmental sectors.
Notable Exceptions and Special Cases
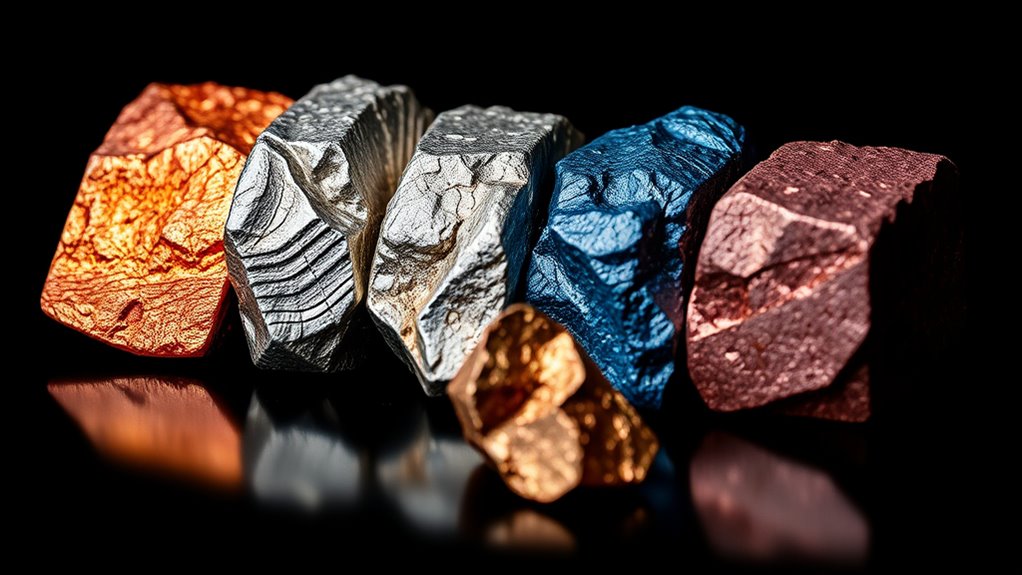
Have you ever wondered why some transition metals defy their typical electron configurations or oxidation states? You’ll find that elements like chromium (Cr) and copper (Cu) deviate from standard filling rules, favoring half-filled or full d-subshells for stability. The stability of half-filled and fully-filled subshells is a key factor in these deviations. Scandium only shows a +3 state, while zinc mainly exhibits +2, due to their d-orbital configurations. Mercury (Hg) challenges the idea of group 12 metals, with HgF₄ showing a +4 oxidation state. Relativistic effects in copernicium (Cn) suggest it might use d-electrons beyond +2 states. Additionally, some ions like Cu²⁺ achieve stability without complete shells. These exceptions highlight unique behaviors, making transition metals a complex and fascinating group with surprises that challenge traditional electron and oxidation state rules.
Frequently Asked Questions
How Do Transition Metals Differ From Main Group Metals?
You notice that shift metals have more complex properties than main group metals. They often form multiple oxidation states, create stronger covalent bonds, and are more electronegative.
Their d-orbitals enable the formation of stable complexes and catalytic activity. Additionally, they generally have higher melting and boiling points, are better conductors, and resist corrosion more effectively.
These differences stem from their unique electronic configurations and bonding capabilities.
Why Are Some Transition Metals Radioactive?
Imagine a metal that glows with a mysterious, unstable energy—some change metals are radioactive because their nuclei are unbalanced.
You see, when protons and neutrons in these atoms don’t match perfectly, they become unstable and decay over time, releasing radiation. This process transforms the element, making it radioactive.
You encounter these metals in nuclear power, medicine, and environmental studies, where their unstable nuclei become powerful tools or pose health risks.
How Do Transition Metals Contribute to Biological Systems?
You see, shift metals are crucial in biological systems because they act as cofactors for enzymes, boosting their activity. They help with oxygen transport, like iron in hemoglobin, and facilitate electron transfer for energy production.
Additionally, metals like zinc stabilize DNA-binding proteins, influencing gene regulation. Their versatility enables them to participate in processes like nitrogen fixation and signal transduction, making them essential for your body’s normal functioning.
What Role Do Transition Metals Play in Renewable Energy?
You see, shift metals are crucial in renewable energy because they enhance efficiency and durability. They serve as catalysts in fuel cells, making hydrogen energy production more effective.
Metals like nickel and zirconium are used in electrolyzers, helping produce clean hydrogen. They also support battery performance and electrical conductivity, which are essential for wind turbines and electric vehicles.
As demand grows, recycling and sustainable practices become increasingly important.
How Are Transition Metal Complexes Used in Medicine?
You might think medicine is straightforward, but the truth is, shift metal complexes hold remarkable power. They’re used as versatile drugs, fighting cancer, infections, and inflammation. With their ability to target specific molecules and undergo unique reactions, they’re essential in modern therapy.
From platinum-based cancer drugs to zinc’s antiviral properties, these complexes continue to evolve, promising safer, more effective treatments—yet challenges remain, driving ongoing research and innovation.
Conclusion
You now see how transition metals are essential in our daily lives, from building materials to catalysts. Did you know that over 40 elements are classified as transition metals? Their unique properties, like variable oxidation states and ability to form colorful compounds, make them fascinating. Whether in jewelry, electronics, or industrial processes, these metals play a vital role. So next time you see a metal object, remember, it’s likely a transition metal making it special.