Advances in electrochemical energy storage are revolutionizing how you store and use renewable energy. Innovations like solid-state and sodium-ion batteries reduce reliance on critical minerals, improve safety, and cut costs. New materials such as graphene and conductive polymers boost performance and lifespan. Flow batteries and hybrid systems expand long-duration options, while policy support accelerates deployment worldwide. To explore how these breakthroughs are shaping a cleaner, more resilient energy future, keep exploring further.
Key Takeaways
- Emerging battery chemistries like sodium-ion and solid-state batteries enhance safety, reduce costs, and improve energy density for long-duration storage.
- Innovations in supercapacitor materials, such as graphene composites and conductive polymers, significantly boost charge capacity and cycle life.
- Flow batteries and hybrid systems offer scalable, durable solutions for large-scale renewable energy integration.
- Recycling and use of abundant elements lower environmental impacts and resource reliance in battery manufacturing.
- Policy incentives and market trends accelerate development and deployment of advanced electrochemical storage technologies.
Breakthroughs in Lithium-Ion Battery Cost and Chemistry
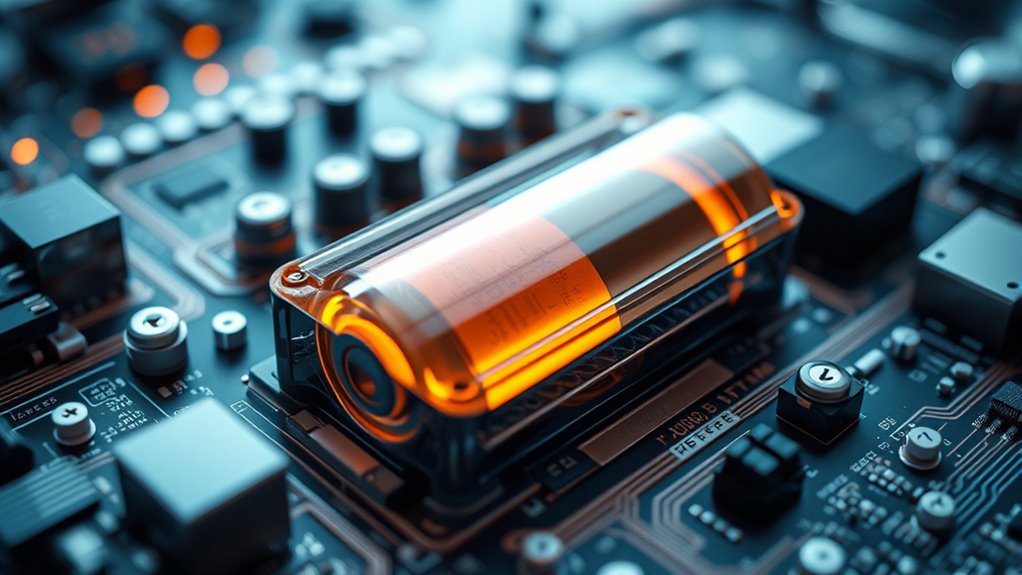
Recent advances in lithium-ion battery technology have driven significant reductions in cost and improvements in chemistry, making energy storage more affordable and efficient. In 2024, prices dropped 20% year-over-year to about $115 per kWh, thanks to overcapacity and the rising adoption of lithium iron phosphate (LFP). This trend is expected to continue, with a forecasted $3 per kWh reduction in 2025, though material cost pressures will slow progress. By 2026, prices could decline by 50% from 2023 levels, reaching around $80 per kWh. Meanwhile, global cell capacity hits 3.1 TWh, exceeding annual demand by over two and a half times. Despite shrinking margins, innovations like cell-to-pack designs and increased silicon anode use are boosting efficiency and reducing structural costs across manufacturing. Metal prices are expected to increase in the longer term, which could influence future battery costs.
Emerging Electrochemical Technologies for Long-Duration Storage
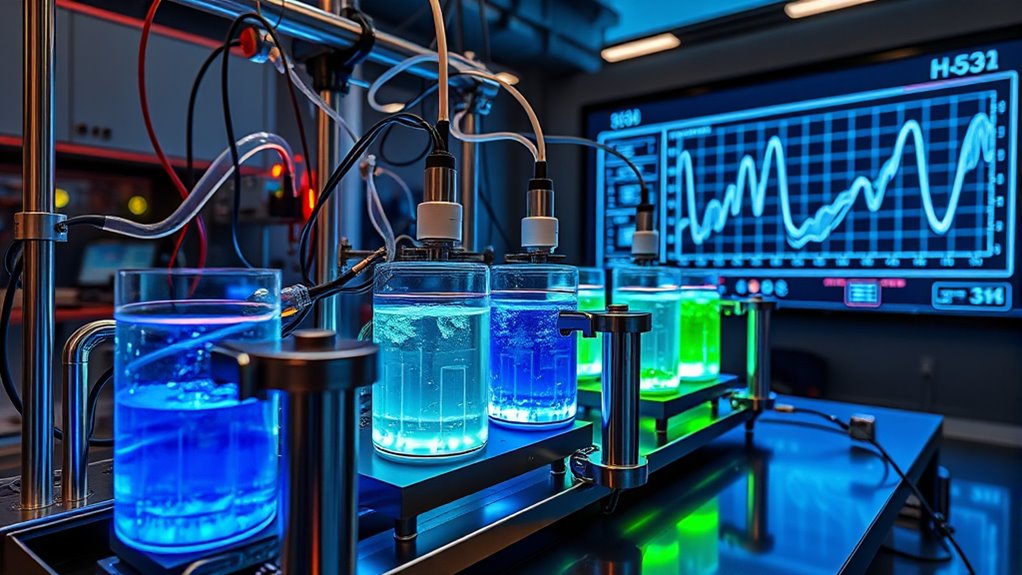
As advancements in lithium-ion batteries continue to lower costs and boost efficiency, the need for long-duration energy storage solutions becomes more pressing to support renewable integration and grid stability. Emerging flow battery technologies, like vanadium redox systems, offer scalability for large solar and wind farms, while organic flow batteries provide lower-cost, sustainable options with similar efficiency. Hybrid designs combining zinc-bromine or iron-salt chemistries improve energy density and cycle life, and modular deployment lets you scale from kilowatts to multi-megawatt systems. Compressed Air Energy Storage hybrids, such as isothermal CAES, reduce energy losses and reach efficiencies of 60-70%, with underground salt caverns supporting large-scale, long-duration discharge. Sodium-ion batteries and solid-state designs also advance, enhancing safety, cost, and energy density for grid applications. Significant progress in material science continues to drive innovation in these emerging electrochemical storage technologies, enabling more durable and affordable systems.
Innovations in Supercapacitor Materials and Performance
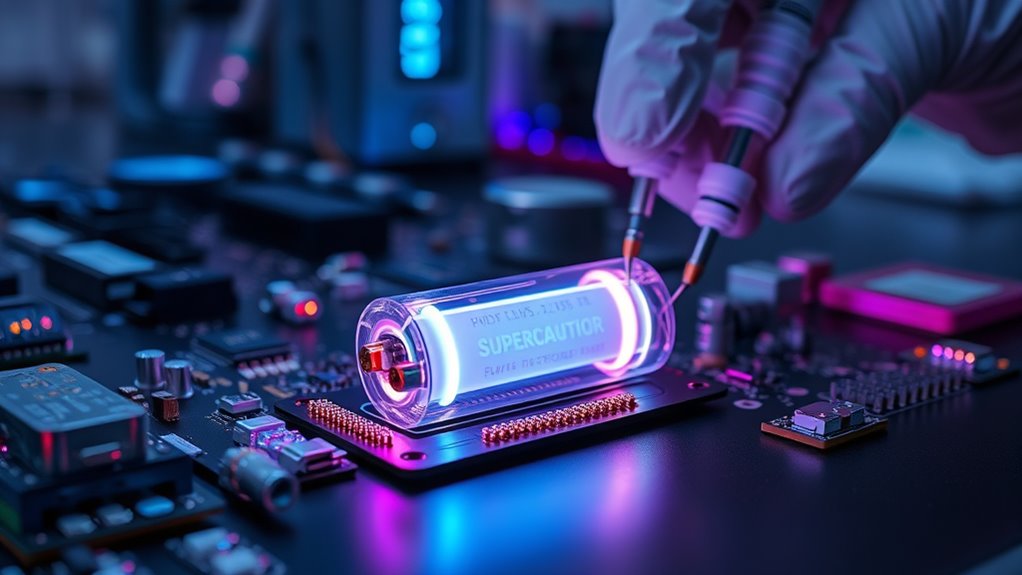
Innovations in supercapacitor materials are driving remarkable performance enhancements, enabling faster charging, higher energy density, and improved durability. Graphene-based composites now offer high conductivity and surface area, with graphene-PEDOT electrodes reaching capacitances over 4600 mF/cm²—almost ten times higher than conventional materials—and enduring over 70,000 charge cycles. Conductive polymers like PEDOT nanofibers achieve 100 times better conductivity and quadruple electrochemically active areas, making them ideal for flexible electronics and harsh environments. Market-wise, activated carbon remains dominant due to low cost, but graphene and hybrid materials push performance boundaries. These advances lead to lighter, more efficient supercapacitors suitable for rapid power delivery in applications from grid stabilization to wearable devices, notably expanding the scope and reliability of energy storage solutions. Efforts to scale production are also accelerating, aiming to make these advanced materials more commercially viable and widely accessible.
Impact of Policy Incentives and Regulations on Energy Storage Adoption

Policy incentives and regulations are substantially shaping how energy storage systems are adopted across markets. The federal tax credit expansion through the IRA offers a 30% credit for standalone storage, with direct pay options for tax-exempt entities and bonuses for domestic content and low-income communities. State programs further support storage adoption via demand response, capacity payments, rebates, and VPP incentives, while renewable standards increasingly include storage carve-outs. Regulatory developments like FERC Order 2023 and wholesale market updates accelerate project interconnections and market participation, though tariff restructuring poses risks to economics. Financial investments surged to $17.6 billion in 2024, driven by corporate PPAs and expanded tax equity. Global supply chain disruptions These policies and regulations create a favorable environment, boosting deployment, domestic manufacturing, and innovative storage applications.
Market Trends Driving Growth in Grid-Scale and Emerging Markets
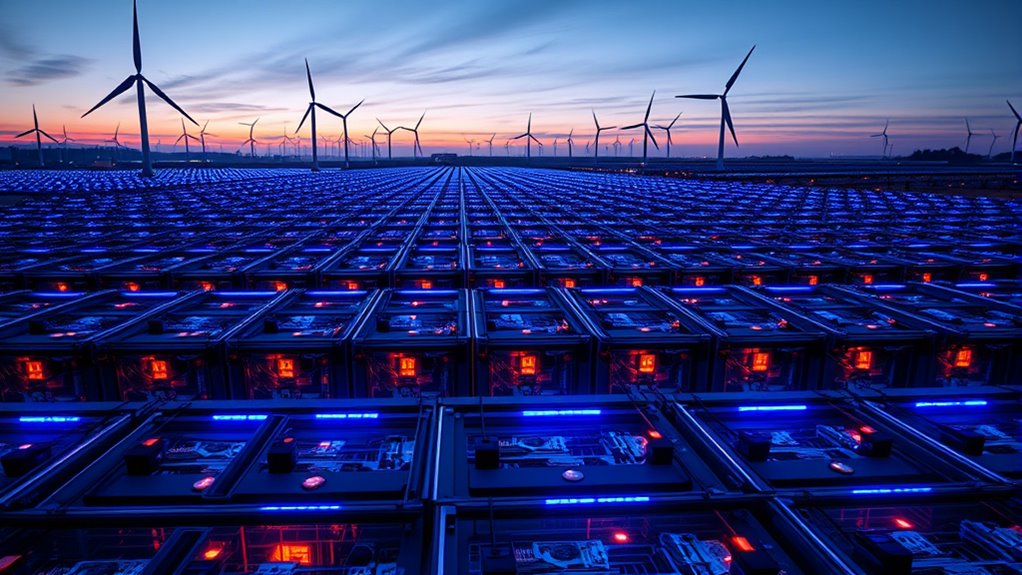
The growth of the global electrochemical energy storage market is accelerating rapidly, driven by the increasing need for grid stability and renewable energy integration. You’ll see this reflected in the market’s rise from a $50 billion valuation in 2025 to an expected $150 billion by 2033, with a 15% CAGR. Lithium-ion batteries dominate grid-scale projects due to their performance, while flow batteries and advanced lead-acid systems are gaining ground. Emerging markets like Saudi Arabia, China, and India are leading expansion efforts, fueled by investments in solar and wind. As technology improves and costs decline, demand for energy storage solutions in these regions continues to surge. The global market size is forecasted to reach USD 569.39 billion by 2034, and supportive policies and innovations in energy management are propelling this growth, creating opportunities across diverse applications worldwide.
Interdisciplinary Advances Connecting Energy Storage With Other Fields
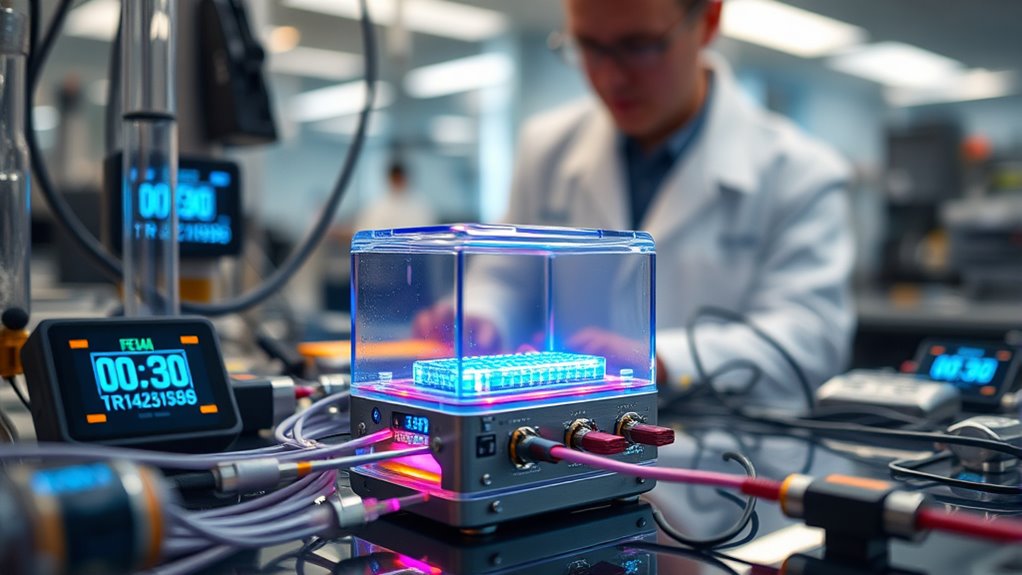
Interdisciplinary collaboration plays a crucial role in advancing electrochemical energy storage, bringing together experts from materials science, electrochemistry, and engineering to develop innovative solutions. You work with diverse teams to create new materials that boost performance and efficiency. Electrode engineering innovations improve device capabilities, while techno-economic models like BatPaC help you evaluate costs and scalability. In flexible energy storage, you develop gel electrolytes and electrode materials suitable for wearable tech and portable electronics. Integration with structural materials leads to multifunctional devices, combining energy storage with mechanical strength—ideal for aerospace and automotive uses. Additionally, advances in energy conversion technologies, like batteries and fuel cells, rely on cross-disciplinary knowledge of thermodynamics, phase transformations, and system scalability. Collaboration across disciplines pushes the boundaries of what energy storage can achieve across multiple fields.
Sustainability and Material Reduction in Next-Generation Batteries
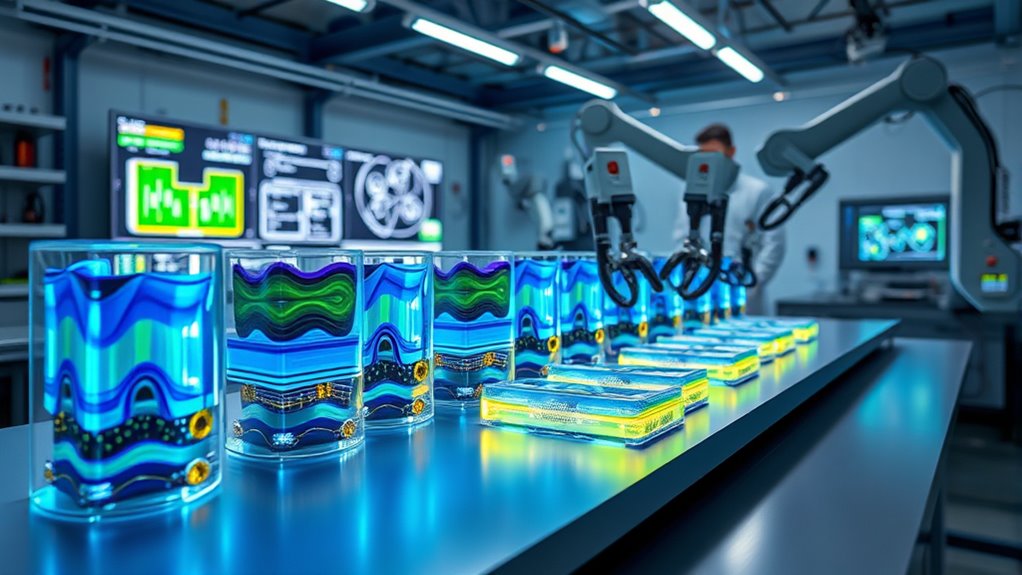
Advancing electrochemical energy storage demands not only innovative performance improvements but also a strong focus on sustainability and reducing material use. You should consider how the growing demand for eco-friendly battery materials, valued at USD 52.99 billion in 2025, aims to lower environmental impacts. Developing batteries with abundant elements like sodium cuts reliance on critical minerals, easing mining concerns. Recycling old batteries recovers materials, reducing greenhouse emissions and conserving resources like lithium, cobalt, and nickel. Alternative materials, such as manganese-rich or cobalt-free options, are becoming more cost-effective and environmentally friendly. Innovations like sodium-ion and solid-state batteries further minimize material needs. These efforts support a shift to sustainable energy storage, decreasing costs, and lowering ecological footprints while meeting the rising global demand for greener solutions.
Future Outlook: Integrating New Technologies for a Resilient Energy System
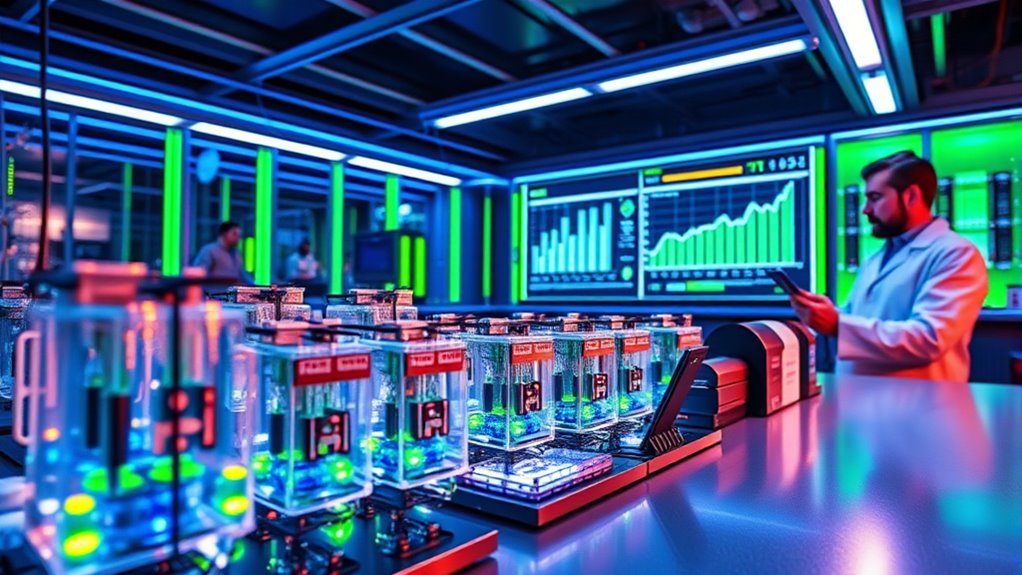
Integrating new electrochemical technologies is essential for building a resilient energy system capable of supporting a sustainable future. You can leverage solid-state batteries to improve safety, energy density, and lifespan, making energy storage more reliable. Lithium Iron Phosphate (LiFePO4) modules enable stable, scalable grid applications, while innovative electrolytes boost performance and longevity. Scalable manufacturing techniques facilitate mass production, lowering costs and expanding deployment. Hybrid systems combining various storage methods optimize performance and reliability, helping stabilize grids and integrate renewable sources like wind and solar. Emerging technologies such as CAES, supercapacitors, and SMES provide rapid response and large-scale storage options. By investing in policy support, research, and infrastructure, you can help overcome scalability and cost challenges, creating a resilient, sustainable energy future.
Frequently Asked Questions
How Do Electrochemical Energy Storage Systems Impact Grid Stability and Resilience?
You see, electrochemical energy storage systems boost grid stability by providing rapid frequency regulation, voltage control, and reactive power support. They quickly respond to supply-demand changes, mitigate renewable intermittency, and emulate inertia, helping prevent blackouts. Additionally, they enable capacity deferral, reduce congestion, and support grid restoration. Their resilience is essential for future energy systems, though cybersecurity and seasonal limitations pose ongoing challenges to maximize their full potential.
What Are the Safety Concerns Associated With Emerging Battery Chemistries?
Imagine a double-edged sword: emerging battery chemistries promise higher energy but come with safety risks. You need to contemplate issues like thermal instability, polysulfide dissolution, and material scarcity. While advancements like solid-state electrolytes and nanoengineering improve safety, scaling up and maintaining consistency remain hurdles. Staying aware of these concerns helps you better understand the delicate balance between innovation and safety in battery technology.
How Does Recycling and Second-Life Use Influence Battery Sustainability?
Recycling and second-life use considerably boost battery sustainability by reducing waste and conserving critical materials. You prevent batteries from ending up in landfills and lower greenhouse gas emissions compared to mining new resources. Repurposing retired EV batteries extends their life, cuts costs, and supports a circular economy. However, challenges like collection gaps, standardization issues, and high recycling costs need addressing to maximize these benefits and ensure a sustainable future.
What Role Does Artificial Intelligence Play in Optimizing Energy Storage Management?
You see, AI plays a vital role in optimizing energy storage management by making smart decisions in real-time. It helps you determine the best charge and discharge cycles, predicts maintenance needs, and maximizes revenue through market participation. AI also coordinates storage with renewable sources, stabilizes the grid, and reduces costs. By continuously learning from data, it guarantees your energy systems operate efficiently, reliably, and sustainably.
How Are New Materials Advancing the Charging Speed of Supercapacitors?
Picture supercapacitors as racecars, zooming at lightning speed. New materials act as turbochargers, boosting their charging speeds. Nanostructured materials, like molybdenum trioxide, create fast lanes for ions, while graphene acts as a magnetic highway, whisking ions along quickly. Composite electrodes and mesoporous niobium oxide serve as high-performance engines, making supercapacitors charge faster and deliver power swiftly, perfect for energy-hungry devices and rapid energy needs.
Conclusion
As you explore the evolving landscape of electrochemical energy storage, you’ll find innovation invigorating industries and inspiring integration. From breakthrough batteries to bold, boundary-pushing materials, progress propels progress. By embracing advancements, encouraging innovations, and enacting effective policies, you can help foster a future where flexible, fast, and functional energy solutions fuel sustainable, secure, and societal success. Together, these transformative trends turn today’s challenges into tomorrow’s triumphs.