Organic chemistry focuses on carbon-containing compounds, like those found in plants, animals, and fuels, with complex structures and covalent bonds. Inorganic chemistry deals with minerals, metals, and non-carbon substances, typically featuring simpler ionic bonds. The two fields differ in sources, structures, reactions, and applications—organic’s versatility in biological systems contrasts with inorganic’s stability and uses in materials and catalysts. Exploring further can help you understand how these differences impact real-world chemistry.
Key Takeaways
- Organic chemistry studies carbon-containing compounds primarily derived from living organisms, while inorganic chemistry focuses on minerals, metals, and non-carbon substances.
- Organic molecules feature covalent bonds, complex structures like chains and rings, whereas inorganic compounds often have simpler ionic or crystalline arrangements.
- Organic chemistry emphasizes biological processes and synthetic pathways, whereas inorganic chemistry deals with mineral structures, catalysis, and materials.
- Organic compounds are typically volatile, flammable, and water-insoluble; inorganic compounds tend to be stable, water-soluble, and form crystalline solids.
- The analysis methods and bonding types differ: organic chemistry relies on covalent bonds and spectroscopic techniques, while inorganic chemistry often involves ionic bonds and crystallography.
Defining the Scope of Organic and Inorganic Chemistry
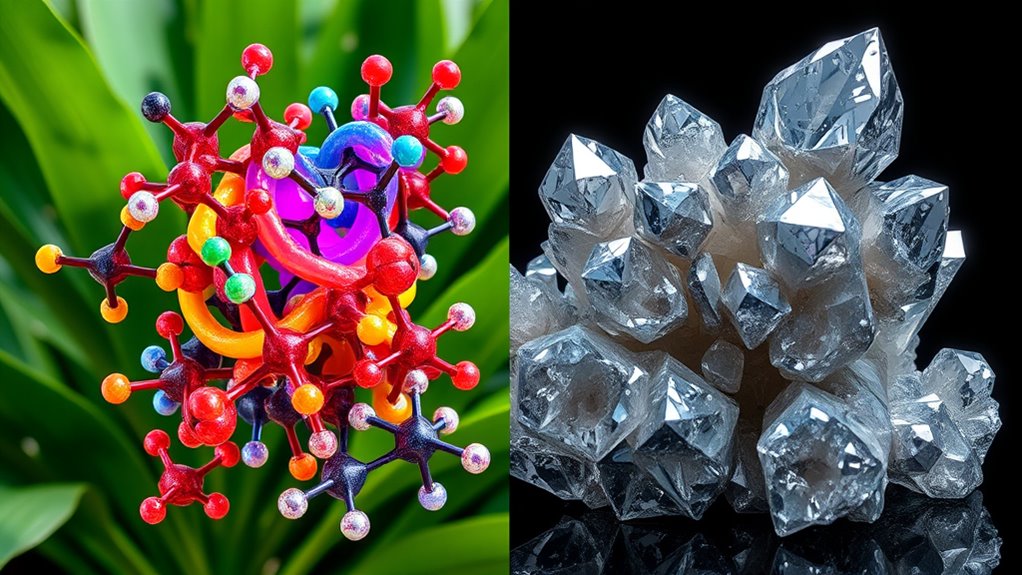
Understanding the scope of organic and inorganic chemistry helps you appreciate how each field specializes in different types of compounds and applications. Historically, organic chemistry developed from the study of carbon compounds and was initially focused on natural substances. Educational approaches emphasize its broad scope, including the structure, properties, and reactions of organic molecules. In contrast, inorganic chemistry’s development centered on minerals, metals, and non-carbon compounds. Its educational methods highlight their roles in geochemistry, catalysis, and materials science. Both fields have distinct focuses but often overlap in areas like materials and catalysis. Recognizing their historical development and educational approaches clarifies how each discipline addresses different chemical questions, shaping their unique contributions to science and industry. Additionally, the distinct methods of analysis used in each field reflect their different focuses and applications.
The Role of Carbon in Differentiating the Fields
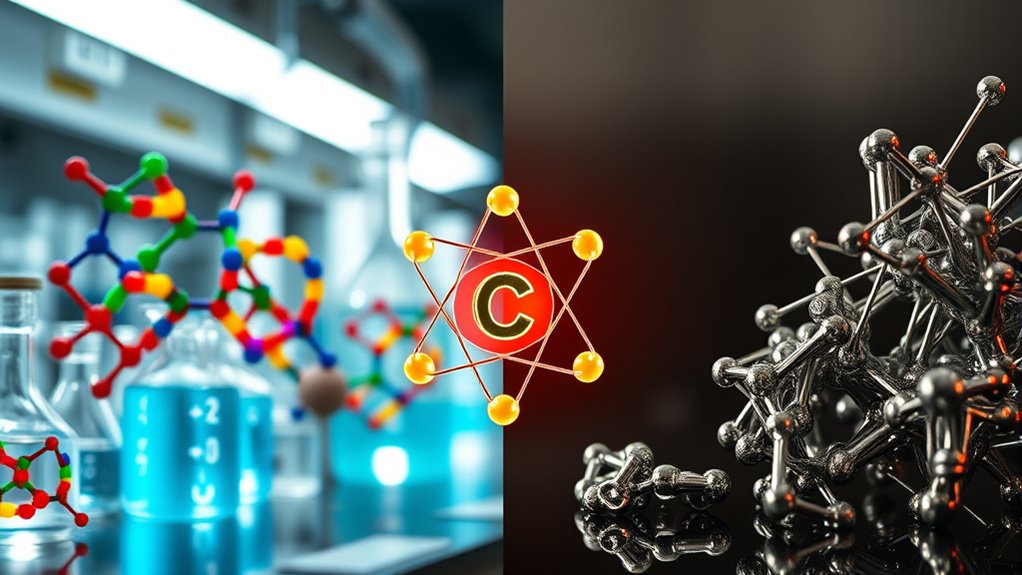
The presence of carbon is the key factor that distinguishes organic chemistry from inorganic chemistry. Carbon’s ability to hybridize into sp³, sp², and sp orbitals allows it to form diverse molecular shapes, which influence functional group reactivity. These groups—like hydroxyl or carboxyl—drive the chemical behavior and reactivity of organic molecules. In organic chemistry, this versatility enables complex structures such as long chains and rings, essential for biological systems and materials. In contrast, inorganic compounds typically lack this hybridization flexibility and functional groups, relying more on ionic interactions. Carbon’s unique bonding capabilities underpin the structural complexity and reactivity that set organic chemistry apart from inorganic chemistry, where bonding is often simpler and less varied. The ability of carbon to form four covalent bonds also allows for an immense variety of stable compounds, making it fundamental to life and many synthetic materials. The diversity of carbon compounds contributes significantly to the development of synthetic materials in modern technology and industry.
Types of Chemical Bonds Commonly Found
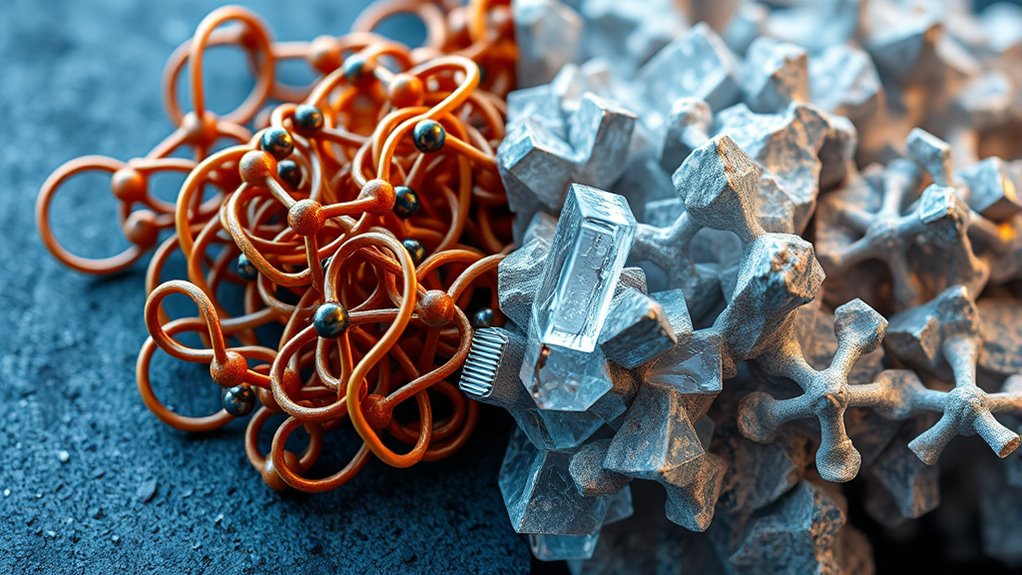
Chemical bonds are the forces that hold atoms together, forming the building blocks of all chemical compounds. When you explore bond formation, you’ll see that ionic bonds occur between metals and non-metals through electron transfer, creating oppositely charged ions. These compounds often have high melting points and are brittle, typical in inorganic salts. Covalent bonds, common in organic chemistry, involve sharing electron pairs, leading to diverse structures like DNA and proteins. They range from single to triple bonds, influencing molecule stability and reactivity. Metallic bonds, found in metals, feature delocalized electrons that enable high conductivity and malleability. Bond strength varies depending on the types of atoms involved and the nature of the bond, this variation further contributes to the structural diversity across both organic and inorganic compounds, shaping the unique properties and behaviors of each class. Additionally, understanding the trustworthiness of AI models is essential as technology advances in fields like chemistry, ensuring safe and reliable application of AI insights. Recognizing different bond types helps in understanding the physical and chemical properties of materials, including their melting points and brittleness.
Origins and Sources of Organic Versus Inorganic Compounds
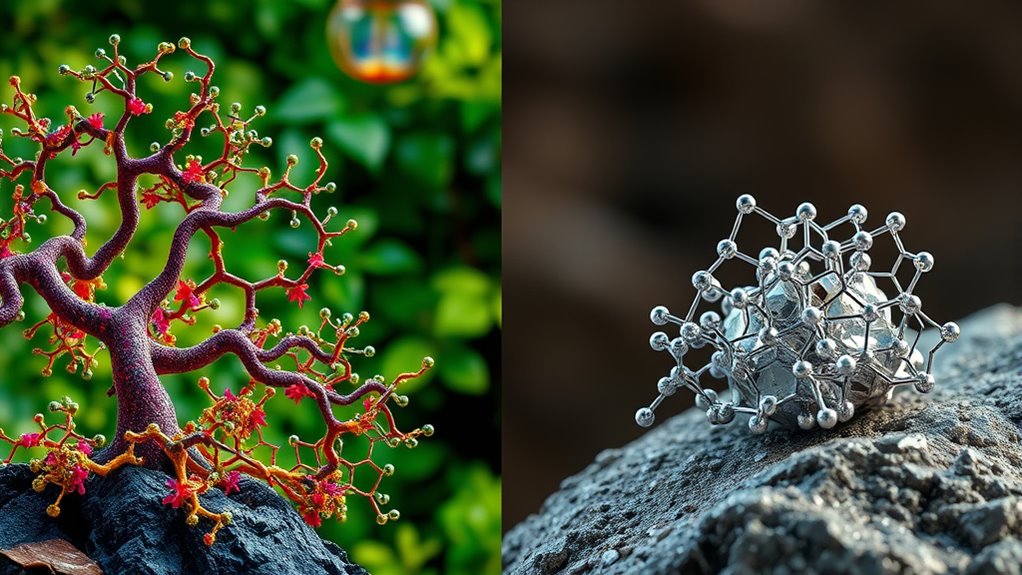
Organic compounds primarily originate from living organisms, forming the basis of biological processes and structures. Historical discoveries, like Friedrich Wöhler’s synthesis of urea, challenged the idea that organic compounds could only come from life, expanding our understanding of their origins. These compounds are mainly found in plants, animals, and fossil fuels, created through processes like photosynthesis and metabolism. In contrast, inorganic compounds come from non-living sources such as minerals, salts, and atmospheric gases, often formed through geological processes like weathering. Environmental impacts differ as organic compounds can contribute to pollution or biological effects, while inorganic compounds are essential for industrial uses. Both types are fundamental, but their sources reflect their biological or mineral nature, shaping their roles in nature and industry. chemical diversity is evident in the wide range of compounds produced by both biological and non-biological processes, illustrating the complexity of natural and industrial chemistry.
Typical Molecular Structures and Complexity
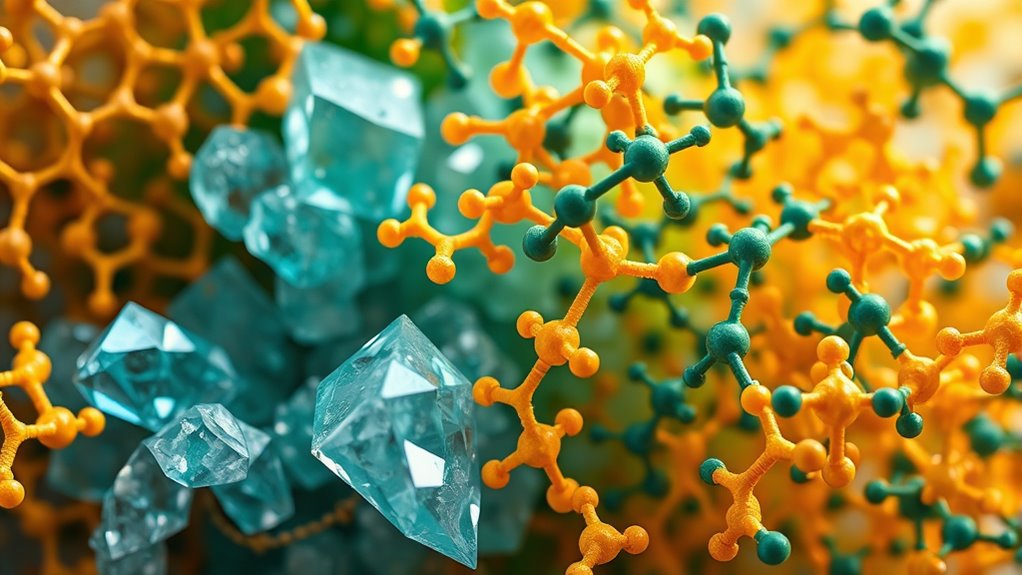
Molecular structures in organic and inorganic compounds differ markedly in complexity and design. Organic molecules exhibit higher molecular complexity, thanks to the versatility of carbon’s bonding ability. Bond diversity is a key factor—carbon forms long chains, rings, and branching structures, creating a wide variety of configurations. This structural flexibility allows for complex functional groups and stereochemistry, adding layers of diversity. In contrast, inorganic compounds tend to have simpler, more uniform structures, often based on ionic bonds and crystalline arrangements involving metals and nonmetals. While inorganic molecules usually lack extensive branching or rings, their structures emphasize ionic interactions over covalent complexity. Understanding these differences helps you grasp why organic compounds are often more structurally intricate and chemically diverse than inorganic ones. The ability of carbon to form stable covalent bonds with many elements is fundamental to the complexity of organic structures.
Acidic Properties and Their Industrial Relevance
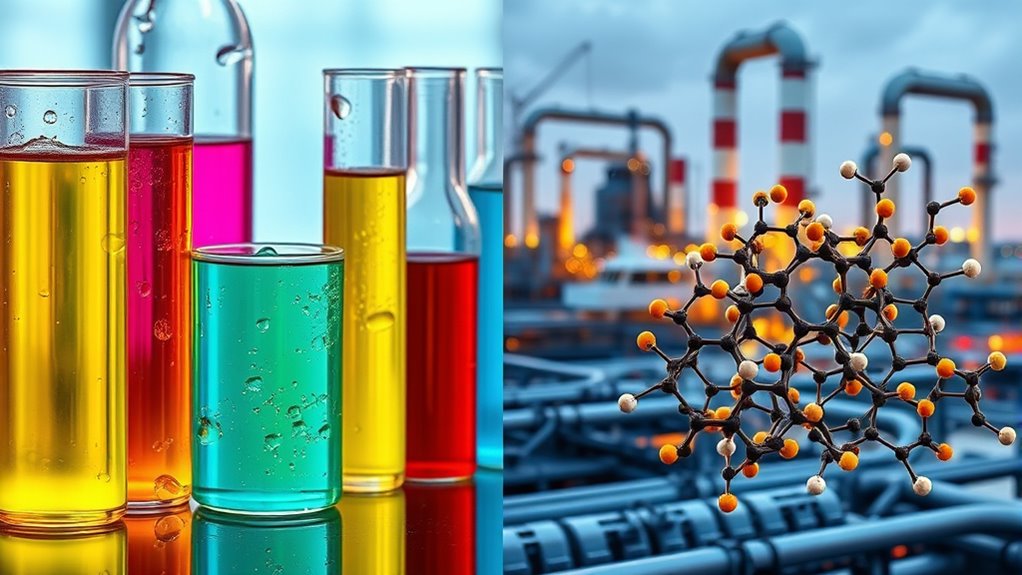
Ever wondered what determines the strength of an acid and why some are more potent than others? Acid strength depends on factors like charge, atom electronegativity, resonance stabilization, and inductive effects. For example, molecules with high electronegativity or electron-withdrawing groups tend to be stronger acids. These properties influence how easily acids dissociate, which is essential for various industrial applications. Strong acids like sulfuric and hydrochloric acids are used in chemical synthesis, electroplating, and wastewater treatment. Organic acids, such as carboxylic acids, find roles in pharmaceuticals, food preservation, and manufacturing. Understanding these acidic properties helps you appreciate their importance across industries, from cleaning products to environmental protection. Their ability to donate hydrogen ions efficiently makes acids indispensable tools in countless industrial processes. Additionally, the filtration systems used in vacuum cleaners can remove residual acid aerosols, helping to maintain safer indoor environments. Recognizing the chemical properties of acids also aids in developing safer handling and storage methods, which is crucial for industrial safety. Moreover, advancements in analytical techniques enable more precise measurement of acid strengths, improving process control and safety. A comprehensive understanding of acid dissociation further enhances the ability to optimize industrial applications and mitigate risks associated with acid use.
Common Reaction Types and Mechanisms
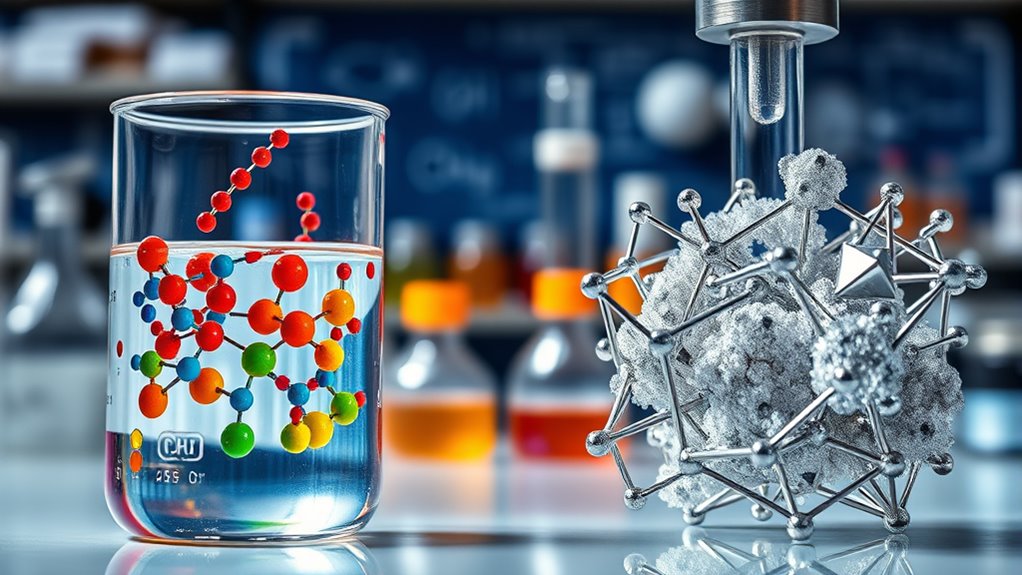
Understanding different reaction types and mechanisms is key to grasping how various compounds transform during chemical processes. Reaction mechanisms reveal the step-by-step bond formation and breaking that drive these changes. In organic chemistry, nucleophilic substitution involves bond formation through SN1 and SN2 pathways, while electrophilic substitution occurs in arenes, forming new bonds via electrophilic attack. Nucleophilic addition is pivotal for aldehydes and ketones, leading to bonds with nucleophiles like Grignard reagents. Inorganic reactions often rely on electron transfer and coordination bonds, especially in redox processes and complex formation. Radical substitution involves radical intermediates reacting with halogens. Recognizing these mechanisms helps you predict reaction outcomes, understand reactivity patterns, and appreciate the distinct ways bonds form across organic and inorganic systems. Additionally, the increased interest in alternative investments such as gold IRAs highlights the importance of understanding different financial mechanisms, much like understanding various chemical reactions. For example, natural materials like wood and stone are frequently used in farmhouse bedroom decor to evoke authenticity and rustic charm. Furthermore, reaction pathways in inorganic chemistry often involve electron transfer steps that are crucial for catalyst design and material synthesis. A solid knowledge of resources and tools can also improve the efficiency of experimental procedures and outcomes.
A thorough grasp of reaction mechanisms also supports the development of new catalysts that enhance industrial processes, making it a vital area of study.
Factors Influencing Stability and Durability
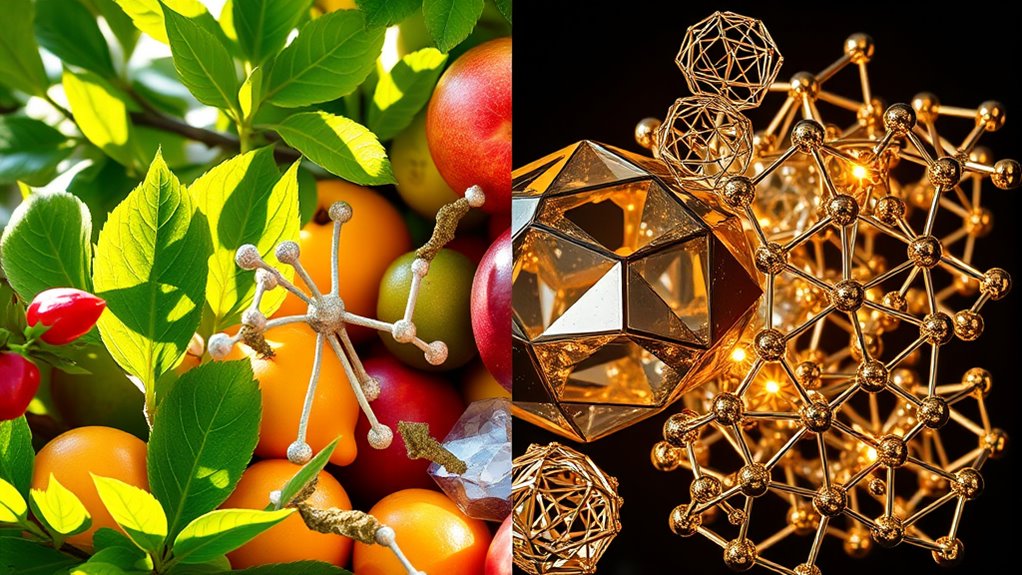
Factors that influence the stability and durability of compounds depend on their chemical structure, bonding, and external conditions. Environmental impact plays a pivotal role, as exposure to oxygen, moisture, and chemicals can degrade organic materials more quickly. Historically, inorganic compounds with ionic bonds and higher thermal stability have shown greater durability, especially under harsh conditions. Molecular shape, cross-linking, and polarity also affect resistance to mechanical and chemical stress. Larger molecules or ions tend to be more durable, while bulky groups can shield against attack. Understanding these factors helps predict how materials will perform over time, considering their origin and development. The presence of resonance stabilization in a molecule can significantly enhance its overall stability by delocalizing charge and reducing reactive sites. Both chemical bonds and external influences shape a compound’s longevity, making stability a key aspect in choosing materials for specific applications.
Applications in Industry and Biological Systems
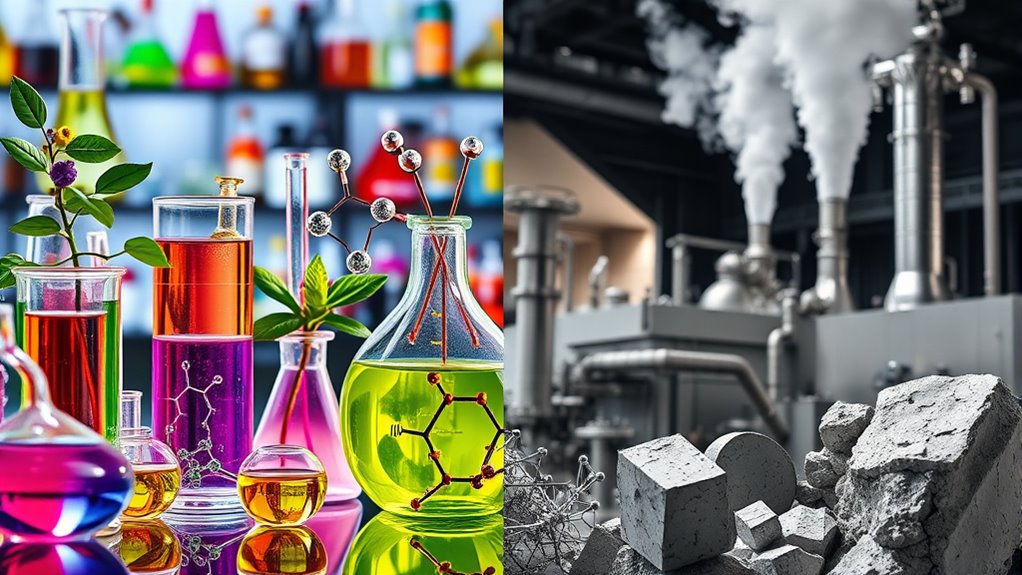
Organic and inorganic compounds serve essential roles across various industries and biological systems, each contributing unique functionalities. Organic compounds are key in producing pesticides, fertilizers, pharmaceuticals, and paints, with their applications driven by their chemical versatility. Inorganic compounds, like sulfuric acid and inorganic ions, are indispensable for fertilizer manufacturing, catalysis, and environmental remediation. Historically, the development of these compounds shaped industrial progress and our understanding of biological processes. Their environmental impact varies; organic compounds from renewable sources can reduce reliance on fossil fuels, though volatile solvents pose toxicity concerns. Inorganic compounds often involve resource-intensive processes but are fundamental for mineral balance, oxygen transport, and biomineralization. Additionally, innovations in sustainable chemistry are leading to more eco-friendly inorganic and organic products. Understanding the environmental impact of these compounds helps guide responsible use and development. Moreover, ongoing research into green synthesis methods aims to minimize ecological footprints associated with both types of compounds. Advances in catalysis are also enhancing the efficiency of chemical reactions, thereby decreasing waste and energy consumption. Furthermore, integrating AI-driven analytics into process optimization is enabling more precise control over chemical manufacturing, reducing waste and improving sustainability. Recognizing these applications highlights how both chemistry branches influence industry, health, and environmental sustainability.
Key Characteristics That Set Organic and Inorganic Chemistry Apart
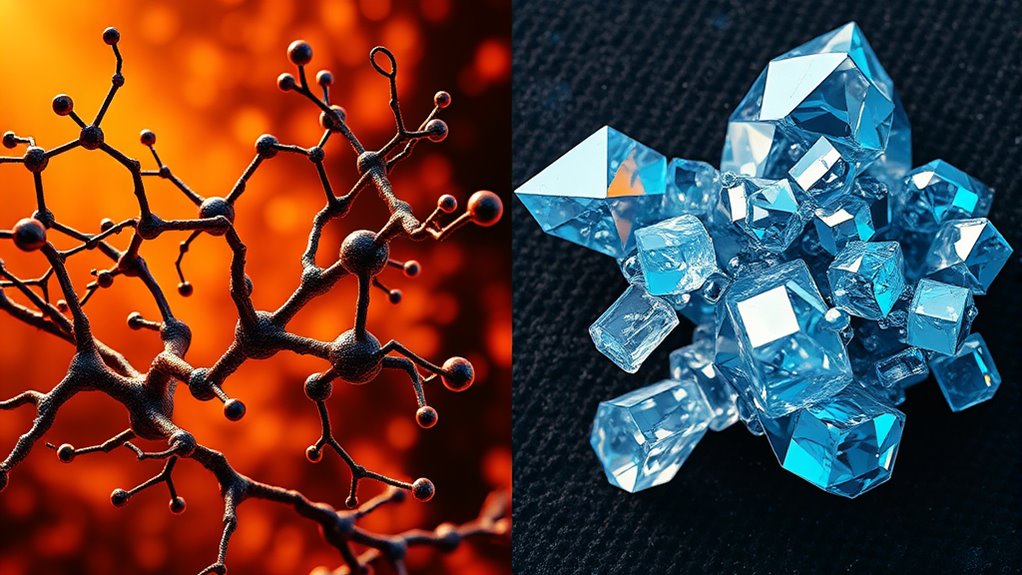
One of the clearest ways to distinguish organic from inorganic chemistry is by examining the presence and role of carbon. Organic compounds always contain carbon, often with hydrogen, and form covalent bonds, making them complex and versatile. Inorganic chemistry mainly focuses on non-carbon elements like metals and minerals, which tend to form ionic bonds. For example, polymer synthesis in organic chemistry involves creating large, chain-like molecules with functional groups, while inorganic chemistry often involves metal coordination complexes that exhibit unique bonding and properties. Organic compounds are typically volatile, flammable, and water-insoluble, whereas inorganic compounds tend to be stable, water-soluble, and conduct electricity. The presence of carbon significantly influences the structure, reactivity, and applications of compounds in both fields. Additionally, the bonding types in each branch greatly impact their chemical behavior and uses. These differences highlight how carbon’s presence influences the structure, reactivity, and applications of compounds in both fields.
Frequently Asked Questions
How Do Organic and Inorganic Chemistry Differ in Their Environmental Impacts?
You should know that organic chemistry tends to have a lower environmental impact because it uses renewable resources and produces biodegradable waste, supporting sustainability practices. In contrast, inorganic chemistry often leads to environmental pollution through toxic waste, greenhouse gases, and non-renewable resource extraction. By choosing organic processes, you can reduce pollution and promote eco-friendly practices, making a positive difference for the environment and future generations.
What Role Do Transition Metals Play in Inorganic Chemistry Compared to Organic Compounds?
You’ll find that transition metals play a crucial role in inorganic chemistry through their formation of coordination complexes, which involve metal ions binding to ligands via d-orbitals. These metals often serve as catalysts in various reactions, thanks to their variable oxidation states and ability to undergo ligand exchange. In contrast, organic compounds typically lack such coordination chemistry, making transition metals essential for many inorganic processes but less common in purely organic systems.
Can Inorganic Chemistry Involve Covalent Bonding Similar to Organic Compounds?
Think of inorganic chemistry as a vast garden, where covalent structures bloom beyond just organic plants. Yes, inorganic chemistry involves covalent bonding mechanisms, just like organic compounds. Atoms share electrons to form stable bonds, creating complex molecules. This sharing isn’t limited to carbon-based molecules; many inorganic compounds, like water or ammonia, rely on covalent bonds. So, covalent bonding is a universal tool, growing diverse structures across both fields.
How Do Organic and Inorganic Compounds Differ in Biological Toxicity?
You can see that organic and inorganic compounds differ in biological interactions and toxicity mechanisms. Organic compounds like DMA V induce lipid peroxidation and deplete GSH, while inorganic arsenic increases ROS and reduces GST activity. Hybrid molecules often show heightened cytotoxicity due to ligand-metal interactions. These differences influence how each compound affects cells, their bioaccumulation, and long-term toxicity, highlighting the importance of understanding their distinct biological toxicity mechanisms.
Are There Overlaps Between Organic and Inorganic Chemistry in Material Science?
In material science, you’ll find significant overlaps between organic and inorganic chemistry through hybrid materials and synthesis methods. You combine organic molecules with inorganic components, like in metal-organic frameworks or conductive polymers, to develop advanced materials. These synthesis methods enable you to tailor properties for energy storage, catalysis, or environmental applications, blending organic flexibility with inorganic stability. This interdisciplinary approach expands possibilities in creating innovative, functional materials for various technological advancements.
Conclusion
So, now you know that organic chemistry loves carbon’s company while inorganic chemistry is the free spirit exploring all other elements. Whether you’re chasing complex molecules or simple salts, each field has its quirks and charms. Remember, one’s all about life’s building blocks, and the other’s about everything else. So go ahead, impress your friends by casually dropping that you understand the difference — they’ll be dazzled, or at least mildly confused.