Batteries work by converting chemical energy into electrical power through reactions at electrodes. During discharge, the anode undergoes oxidation, releasing electrons, while the cathode accepts electrons through reduction. Electrolytes move ions to balance charge, and separators prevent short circuits. Charging reverses these reactions, restoring the battery’s energy. If you want to understand exactly how these processes work and the latest advancements, there’s more to explore beneath the surface.
Key Takeaways
- Batteries convert chemical energy into electrical energy through reactions at the electrodes.
- Oxidation occurs at the anode, releasing electrons; reduction at the cathode accepts electrons.
- Electrolytes enable ion transfer between electrodes during charging and discharging cycles.
- The voltage depends on the electrochemical potentials of electrode materials.
- Reversing reactions during charging restores the battery’s stored chemical energy.
Basic Principles of Battery Energy Conversion
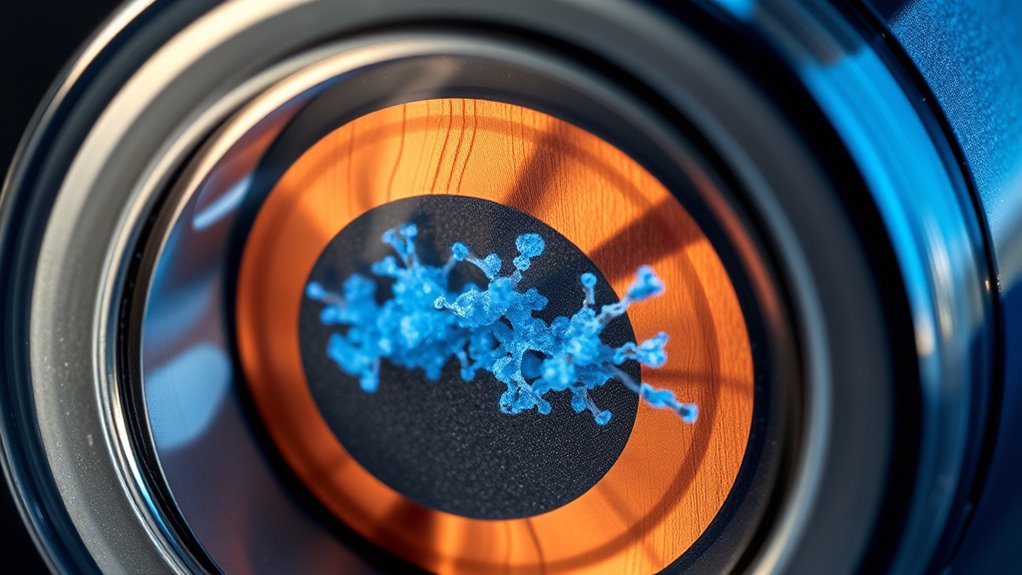
Batteries convert stored chemical energy into electrical energy through electrochemical reactions. You store energy in the form of chemical bonds within electrode materials, which are less stable high-energy reactants like zinc or lithium.
Batteries transform chemical bonds into electrical energy through electrochemical reactions.
When the battery operates, oxidation occurs at the anode, releasing electrons, while reduction happens at the cathode, accepting electrons. Electrolytes facilitate ion transfer to balance charge during these reactions.
The voltage your battery produces depends on the electrochemical potentials of the electrode materials. To maximize efficiency, the system aims to minimize energy lost as heat during the conversion process.
This delicate balance ensures that the chemical potential stored in the electrodes effectively transforms into usable electrical energy, powering your devices reliably and efficiently.
The Role of Electrodes in Chemical Reactions
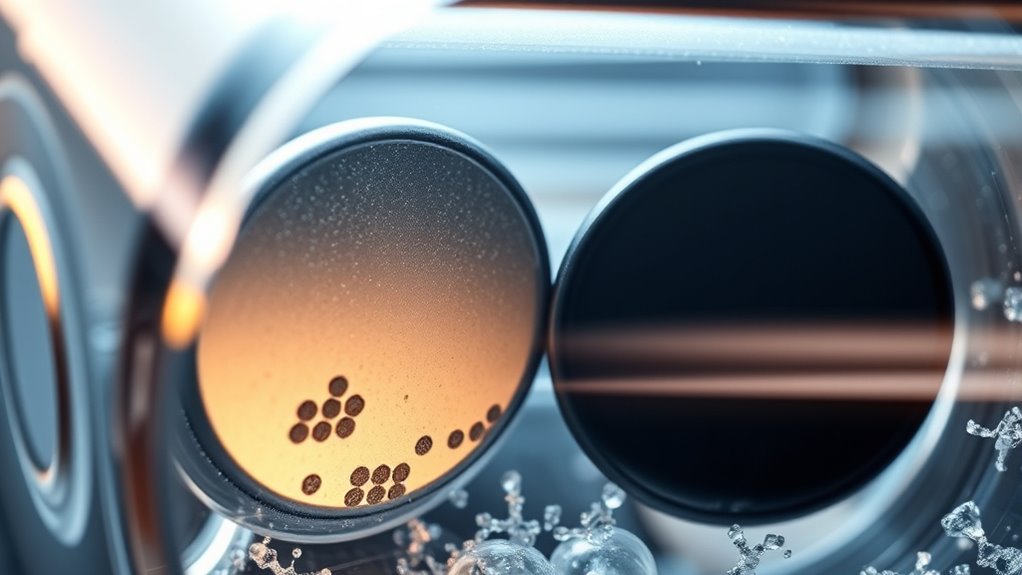
During discharge, electrodes serve as the sites where essential chemical reactions occur, enabling the conversion of chemical energy into electrical energy.
At the anode, oxidation takes place, releasing electrons and generating ions; it acts as the electron source and reduces the material by losing electrons, which travel through the external circuit.
Meanwhile, the cathode absorbs electrons through reduction, accepting them into its structure and maintaining charge balance via ion intercalation or conversion reactions.
The electrode materials, such as lithium or nickel oxide, influence reaction efficiency and energy density.
Surface area and phase stability impact reaction kinetics and long-term performance.
These reactions are driven by electron conduction through current collectors and ion diffusion across interfaces, ultimately powering your device.
Understanding the Electrolyte and Separator Functionality
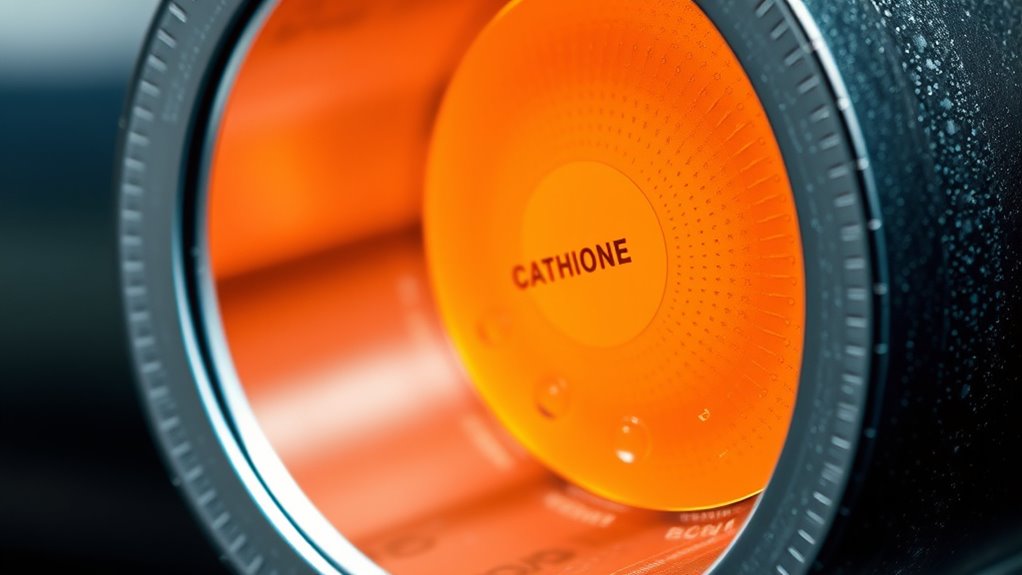
The electrolyte and separator work together to facilitate ion transfer while ensuring safe operation within a battery. The electrolyte, often a liquid with lithium salts like LiPF6 dissolved in organic solvents, allows lithium ions to move efficiently between electrodes during charge and discharge. It permeates separator pores and electrode structures, enabling continuous ion flow. Kia Tuning components like performance batteries can incorporate advanced electrolytes for higher energy density and longevity. The separator acts as a physical barrier that prevents short circuits by stopping electrode contact while allowing ions to pass through its microporous design. Made from materials like polyolefins or ceramics, separators also provide mechanical strength and thermal stability. Some separators feature thermal shutdown layers that melt at high temperatures, halting ion flow to prevent overheating. Together, they enable safe, reliable ion movement essential for battery performance.
The Discharge Cycle: How Energy Is Released
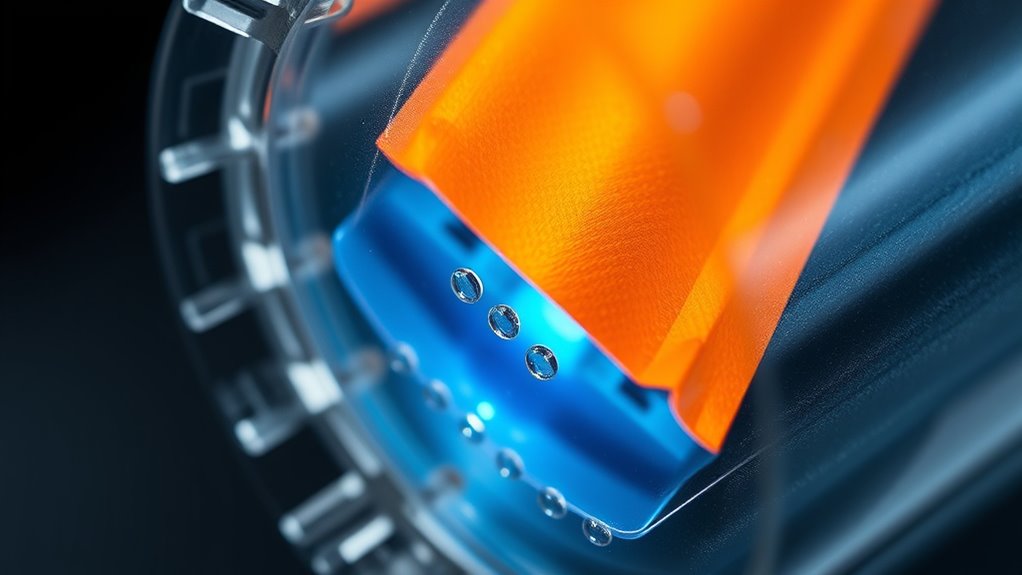
When a device draws power, chemical reactions inside the battery release stored energy by moving electrons through an external circuit. Electrons flow from the anode (negative terminal) to the cathode (positive terminal), generating a voltage difference across the terminals. This electron movement results from electrochemical interactions that convert chemical energy into electrical energy. Proper discharge guarantees maximum performance while preventing damage caused by overdischarging, which can accelerate chemical degradation and shorten the battery’s lifespan. Additionally, maintaining optimal discharge conditions can prolong the overall battery life and ensure safety during operation. Understanding the chemical processes within the battery can help optimize its usage and longevity, especially by adhering to recommended maintenance practices to prevent premature wear. Regular monitoring of battery health can further prevent unexpected failures and extend its effective lifespan, emphasizing the importance of balanced usage to sustain performance over time.
Charging and Reversing Chemical Reactions
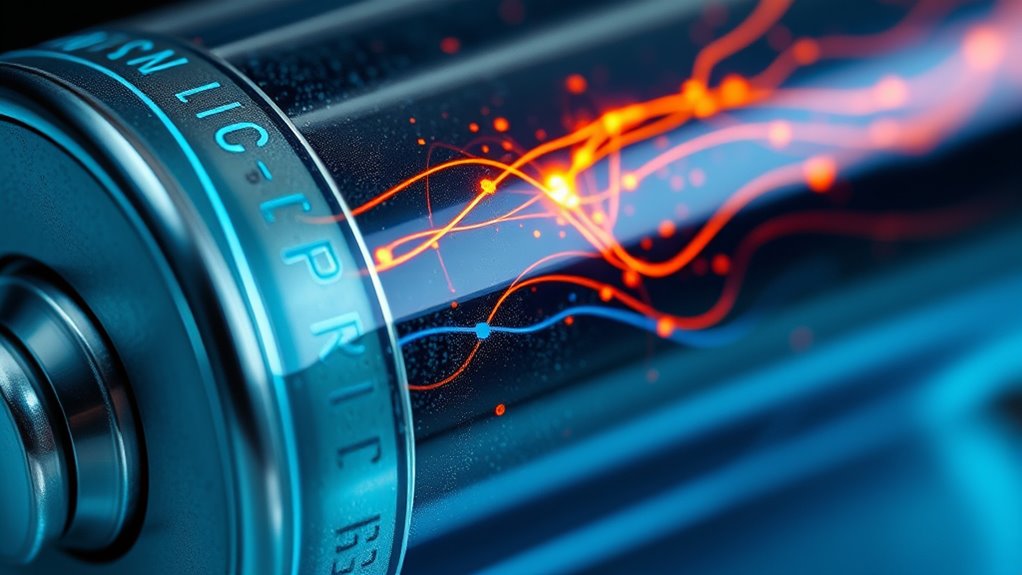
Charging a battery involves applying an external electrical potential that reverses the chemical reactions occurring during discharge. This process uses an external power source to drive oxidation and reduction reactions at the electrodes in the opposite direction. Electrons flow back through the circuit, restoring the active materials to their original states. During charging, ions move through the electrolyte to facilitate these reactions, effectively reversing the formation of compounds like lead sulfate in lead-acid batteries or redistributing lithium ions in lithium-ion batteries. The efficiency of this process depends on factors like temperature and battery age. Proper charging restores the battery’s capacity and prepares it for another cycle of energy release, ensuring its longevity and reliable performance. Additionally, understanding the cycle of breakups and relationship dynamics can help in maintaining emotional well-being, much like maintaining a battery’s health ensures optimal performance. Recognizing the importance of AI safety measures is also crucial to prevent potential vulnerabilities and ensure trustworthy operation. Furthermore, employing appropriate charging protocols can prevent issues such as overcharging or overheating, which are critical for battery safety and longevity. Developing advanced charging techniques can further improve efficiency and extend battery life, especially in high-demand applications.
Innovations and Future Directions in Battery Chemistry
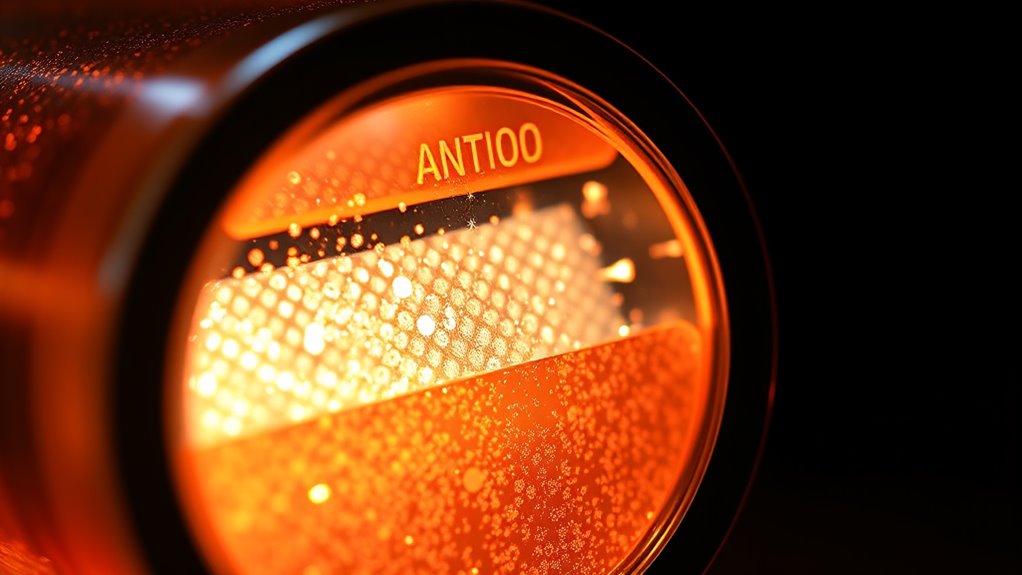
Have you ever wondered how battery technology continues to evolve to meet growing demands for safety, performance, and sustainability? Quasi-solid-state electrolytes are a key innovation, blending non-flammable solid and liquid components to reduce fire risks and improve thermal stability. They offer higher ionic conductivity and longer cycle life, bridging current lithium-ion and future solid-state batteries. Risk assessment is crucial for ensuring these advancements do not introduce unforeseen vulnerabilities. Additionally, the development of advanced electrolyte materials enhances overall battery safety and efficiency. Meanwhile, quantum batteries push boundaries by using quantum state manipulation for faster charging, leveraging controlled dephasing and decoherence. Although promising, scalability remains a challenge for real-world use. Solid-state batteries replace flammable liquids with solid electrolytes, providing higher energy density and faster charging, with commercialization targeted for 2025. Meanwhile, alternative chemistries like sodium-ion and lithium-sulfur batteries diversify supply chains, lower costs, and boost sustainability for the future. Automation in industries continues to accelerate, supporting the development and deployment of these advanced battery technologies.
Frequently Asked Questions
How Does Temperature Affect Battery Performance and Lifespan?
You might notice that higher temperatures temporarily boost your battery’s capacity and efficiency, but they also speed up wear and tear. Elevated heat accelerates chemical reactions, increasing self-discharge and degrading materials over time.
In the short term, performance improves, but long-term, heat shortens your battery’s lifespan. To keep it working well longer, you should manage heat through cooling, insulation, and proper charging practices.
What Causes Battery Capacity to Degrade Over Time?
You might think batteries last forever, but they’re fighting a losing battle against wear and tear. Over time, parasitic reactions eat away at active lithium and electrolytes, while the thickening of the solid electrolyte interphase (SEI) raises internal resistance.
High temperatures, aggressive charging, and deep discharges speed up chemical and mechanical degradation, causing capacity loss. Eventually, these factors chip away at your battery’s ability to hold a charge, leading to failure.
Are There Safety Concerns Related to Battery Chemical Reactions?
You should be aware that chemical reactions inside batteries can pose significant safety risks. Overheating from overcharging, damage, or external heat can trigger thermal runaway, leading to fires, explosions, or toxic chemical leaks.
Flooding or water exposure worsens these hazards, increasing the chance of short circuits or delayed reactions. Handling damaged batteries carefully and following safety protocols helps prevent chemical leaks and minimizes the risk of fires or health hazards.
How Do Different Electrode Materials Influence Voltage Output?
Imagine the voltage as a roaring river, and your electrode materials are the rocks shaping its flow. Different materials with higher electrode potentials create a bigger drop, resulting in more voltage.
You can influence this by choosing electrodes that maximize potential difference. So, your selection directly impacts how powerful your battery feels—more voltage means a more energetic device, powering your gadgets with impressive strength and efficiency.
What Are the Environmental Impacts of Battery Disposal and Recycling?
You should know that improper battery disposal harms the environment through toxic leaks, landfills fires, and resource depletion. Recycling helps reduce these issues by conserving materials, lowering CO₂ emissions, and preventing toxic leaks.
However, low recycling rates, complex designs, and lack of incentives make proper recycling challenging. To protect the environment, support regulations, educate others, and push for better recycling infrastructure and economic incentives.
Conclusion
Now that you see how batteries convert chemistry into power, remember: they store energy quietly like a hidden reservoir, yet release it suddenly like a burst of lightning. The delicate dance of electrodes and electrolytes keeps your devices alive, much like a racecar engine powering a swift ride. As technology advances, your future gadgets will become even smarter and more efficient—proving that behind every device’s glow, there’s a fascinating chemistry working silently in the background.