Recent breakthroughs in nanochemistry include innovations in carbon nanomaterials, nanoscale semiconductors, and energy storage nanomaterials. Advances enable precise nanofilm fabrication, development of functional nanocomposites, and magnetic nanoparticles for cancer therapy. Eco-friendly manufacturing practices and atomic-scale techniques improve synthesis control and sustainability. These innovations open new possibilities for medicine, electronics, and energy. If you’re curious about how these advancements impact various industries, exploring further will reveal even more exciting developments in the field.
Key Takeaways
- Innovative synthesis techniques like in situ nanoparticle generation and vapor-phase methods enable precise control of nanomaterial properties.
- Development of eco-friendly green synthesis methods reduces toxic waste and enhances sustainable nanomaterial production.
- Advanced 3D printing and atomic-scale techniques such as ALD and STM facilitate complex nanostructure fabrication.
- Integration of nanoparticles into biomedical applications improves diagnostics, targeted drug delivery, and cancer therapies.
- Enhanced nanocomposite materials exhibit superior mechanical, thermal, and functional properties for diverse industrial uses.
Innovations in Carbon Nanomaterials
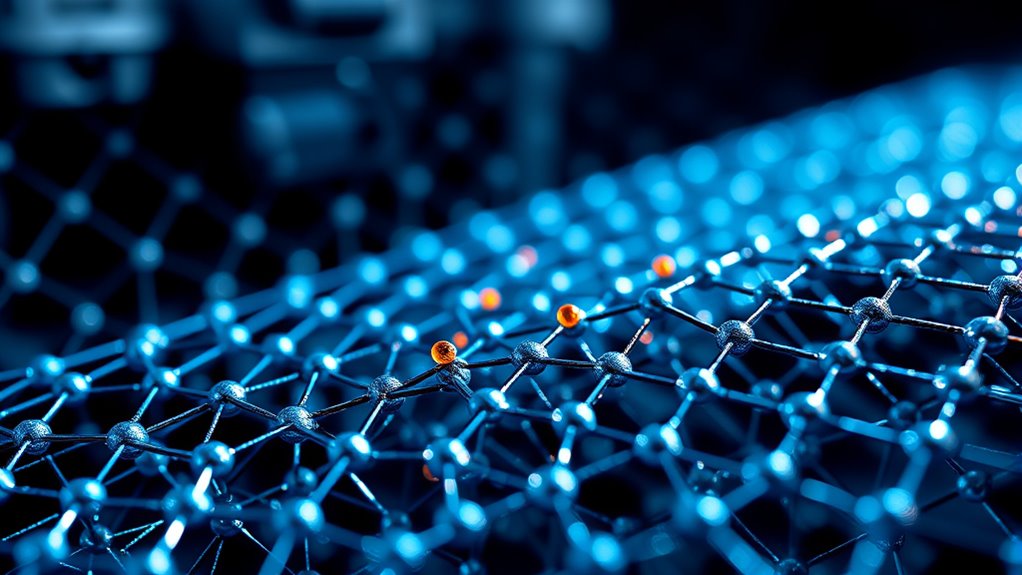
Innovations in carbon nanomaterials have revolutionized multiple industries by leveraging their exceptional properties. You’ll notice that CNT-based coaxial cables weigh only 10% of copper ones, saving you around $40,000 per pound in spacecraft operations. With high thermal and electrical conductivity, EMI shielding, and energy storage capabilities, these materials offer multifunctionality thanks to their high surface-area-to-volume ratio. Manufacturers optimize CNT morphology through electrospinning and chemical vapor deposition, making them ideal for aerospace and defense use. Their lightweight nature reduces fuel consumption and payload costs in space vehicles. Additionally, the high surface-area-to-volume ratio of these nanomaterials enhances their effectiveness in sensors and catalysts, further broadening their industrial applications. The unique electrical properties of CNTs enable their integration into next-generation electronic devices, opening new avenues for innovation. These properties are further supported by ongoing research into nanomaterial synthesis, which seeks to improve the scalability and performance of CNTs for commercial use. Companies like Northrop Grumman now incorporate CNTs into intelligent, multifunctional space systems, pushing the boundaries of material performance. These innovations markedly improve efficiency, durability, and cost-effectiveness across diverse applications, transforming how industries approach design and sustainability.
Advances in Nanoscale Semiconductor Devices
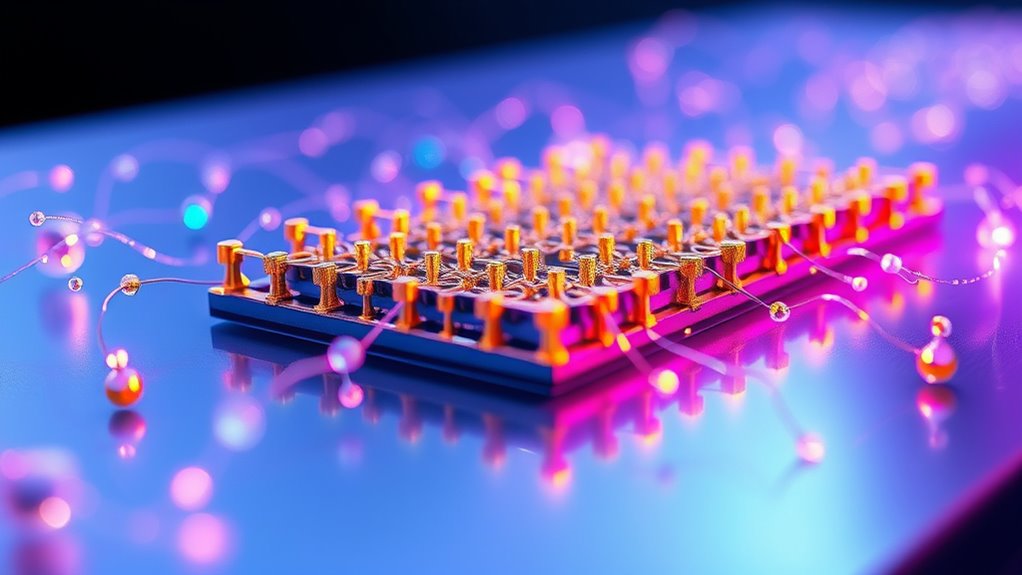
Building on recent breakthroughs in nanomaterials like carbon nanotubes and graphene, the semiconductor industry is pushing the boundaries of device miniaturization. You’ll see new materials like molybdenum disulfide (MoS₂) and other Transition-Metal Dichalcogenides (TMDs) enabling smaller, faster transistors with improved electrical and thermal performance. Advanced interconnects reduce resistance and enhance signal integrity, while novel dielectric materials improve chip reliability. As devices reach the nanoscale, quantum effects become significant, demanding innovative solutions. Manufacturing advances such as Atomic Layer Deposition (ALD) and high-NA EUV lithography allow for finer patterning, supporting sub-2nm chips. Techniques like 3D integration and through-silicon vias (TSVs) boost density and performance without shrinking individual transistors, opening new possibilities for future semiconductor technologies. Moreover, developing scaling techniques is essential to overcome the ongoing scaling challenges and sustain Moore’s Law, driving research into new fabrication methods and materials.
Breakthroughs in Energy Storage Nanomaterials
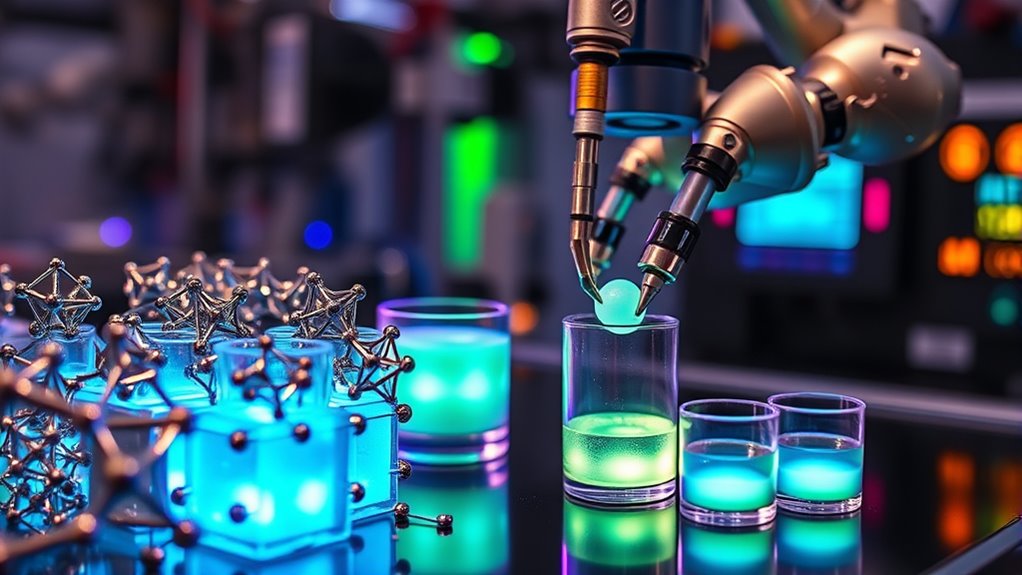
Recent advances in nanomaterials are transforming energy storage technologies by enabling higher capacity, faster charging, and longer cycle life. You’ll find that DyCoO₃@rGO nanocomposites combine rare-earth oxides with graphene, delivering a remarkable 1418 F/g capacitance at 1 A/g and maintaining stability over 5,000 cycles. Its 3D hybrid structure improves electrolyte interaction, boosting ion transport and performance. Nano-engineered anodes, like tin nanoparticle-hard carbon composites, increase lithium and sodium-ion battery energy density while reducing degradation, supporting faster charging and longer-lasting batteries. Silicon nanotube architectures and sulfur-carbon nanocomposites enhance capacity and sustainability. These innovations, alongside nanostructured supercapacitors and solid-state electrolytes, optimize grid-scale and portable energy storage, making renewable power more reliable and extending device lifespans. Nanomaterials continue to play a crucial role in advancing energy storage solutions across various applications, including celebrity lifestyle insights, where premium materials influence high-end designs and durability. Additionally, incorporating the mind-body connection principles from somatic therapy can inspire new approaches to designing more resilient and adaptable energy systems. Moreover, ongoing research into material stability is essential to ensure long-term performance in diverse operating environments. Recent studies also emphasize the importance of regulatory frameworks to facilitate safe and sustainable deployment of these advanced materials in real-world settings. Furthermore, exploring scalable manufacturing methods is vital for transitioning these nanotechnologies from laboratory research to commercial applications.
Development of Functional Nanocomposites
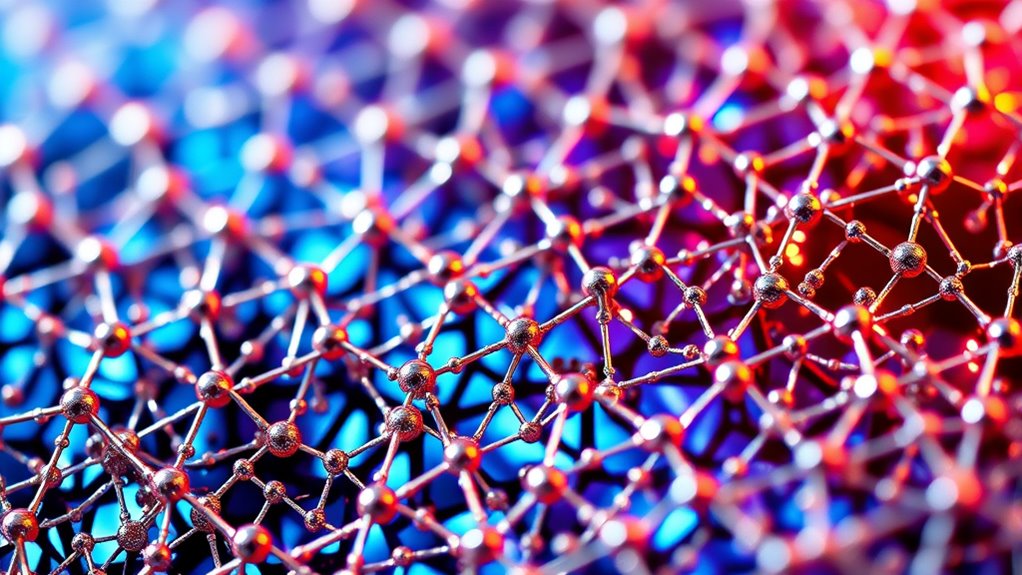
Advances in nanochemistry have paved the way for developing highly functional nanocomposites with tailored properties for diverse applications. You can now synthesize these materials using in situ nanoparticle generation, which creates nanoparticles directly within polymer matrices, or employ sequential infiltration synthesis to grow inorganic nanostructures inside polymers via vapor-phase precursors. Advanced 3D printing techniques incorporate nanoparticles to improve surface properties, while hybrid systems enable controlled dispersion for specific optical or electronic traits. Bio-inspired nanocomposites combine natural polymers with nanoparticles for biomedical compatibility. These methods enhance properties like mechanical strength, thermal stability, flame retardancy, and antimicrobial activity. The ability to precisely control nanoparticle placement and distribution is crucial for optimizing nanocomposite performance and has significantly improved the performance and functionality of nanocomposites in various fields. As a result, nanocomposites find widespread use in biomedical devices, environmental remediation, and high-performance materials, opening new avenues for innovation across industries.
Progress in Nanofilms for Electronic Applications
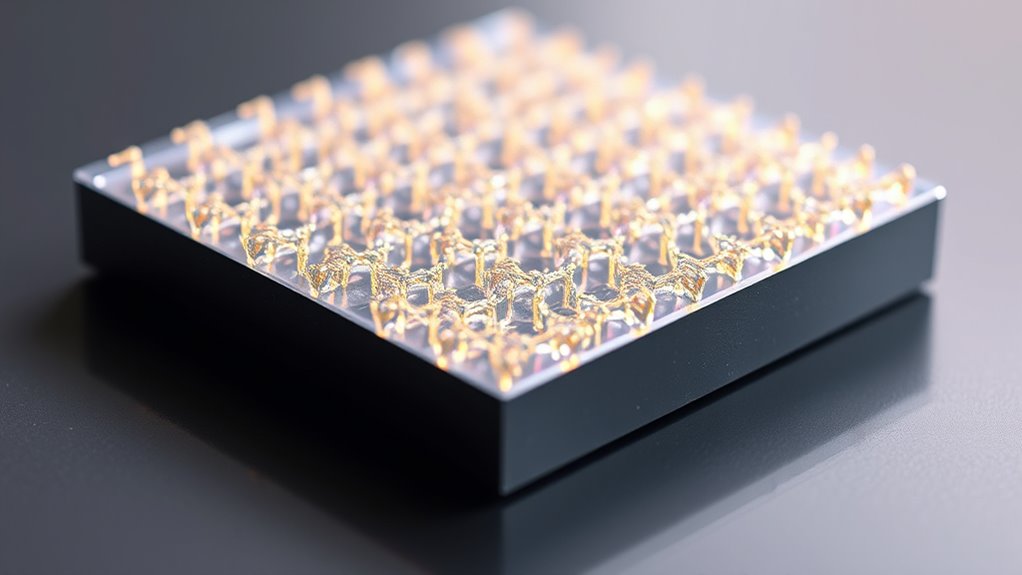
Nanofilms are transforming electronic technologies by providing exceptional control over electrical, optical, and thermal properties. Their enhanced conductivity boosts device performance, while improved optical transparency benefits display technologies. Nanofilms also resist corrosion, protecting components in harsh environments. Depending on their composition, nanofilms can act as efficient thermal barriers or conductors, aiding thermal management. Advances in deposition methods like chemical vapor deposition (CVD) and physical vapor deposition (PVD) enable scalable, cost-effective production for industry. Materials such as graphene, metal oxides, and hybrid polymers deepen the functionality of nanofilms, offering strength, stability, and tunability. The development of new synthesis techniques is crucial for expanding the range of applications and improving material quality in the industry. Additionally, material customization allows for tailored properties suited to specific electronic applications, further expanding their potential uses. These innovations lead to smarter, more durable electronic devices, fueling market growth. As research progresses, nanofilms will become integral in next-generation electronics, balancing performance, sustainability, and manufacturing feasibility. Moreover, ongoing research into nanofilm stability is essential to ensure long-term reliability in various environments, and understanding the environmental impact of nanofilm production is vital for sustainable development. The ongoing exploration of advanced fabrication techniques is opening new avenues for precise control over nanofilm characteristics, enhancing their functionality. Furthermore, understanding the cost-effectiveness of nanofilm production methods is key to facilitating broader industry adoption.
Nanoparticles in Diagnostic Technologies
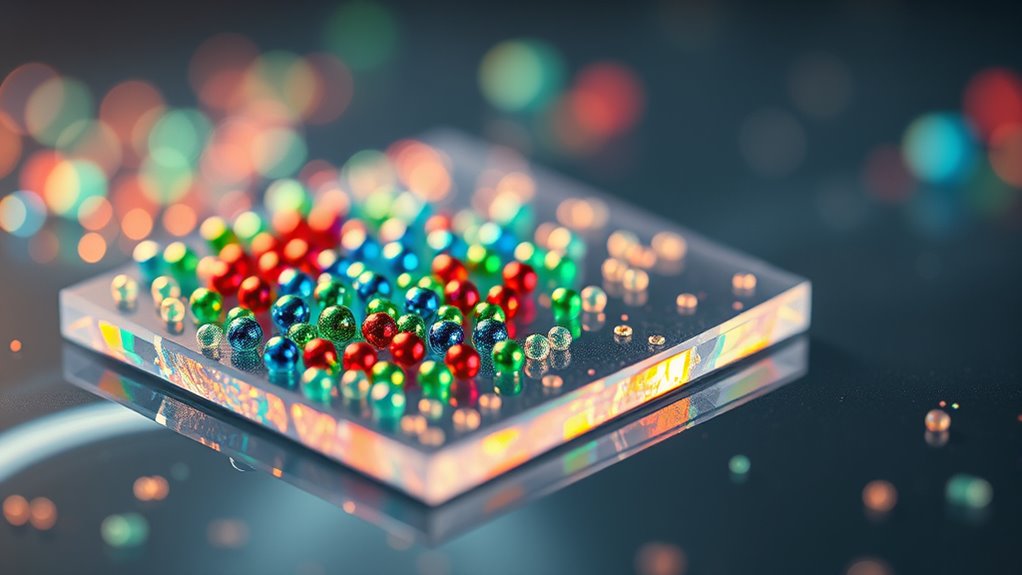
Nanoparticles have revolutionized diagnostic technologies by enabling highly sensitive and specific detection methods. They enhance imaging techniques like MRI and PET scans, improving resolution and accuracy. Gold nanoparticles are used in biosensors to quickly identify viral RNA or proteins, allowing rapid pathogen detection. In genetic disorder diagnosis, nanoparticles facilitate non-invasive liquid biopsies to detect mutations early. Engineered nanoparticles can cross the blood-brain barrier, helping to identify early biomarkers of neurological diseases like Alzheimer’s. They also support personalized medicine by enabling targeted diagnostics and treatments. Nanoparticles improve the sensitivity and speed of biosensors, making portable, point-of-care tools feasible. Additionally, the development of targeted nanoparticle delivery systems is expanding the capabilities of diagnostics and therapeutics. Overall, they make diagnostics faster, more accurate, and more adaptable, transforming how we detect and monitor diseases across various fields. Their ability to enable real-time monitoring further enhances early diagnosis and disease management.
Magnetic Nanoparticles for Cancer Therapy
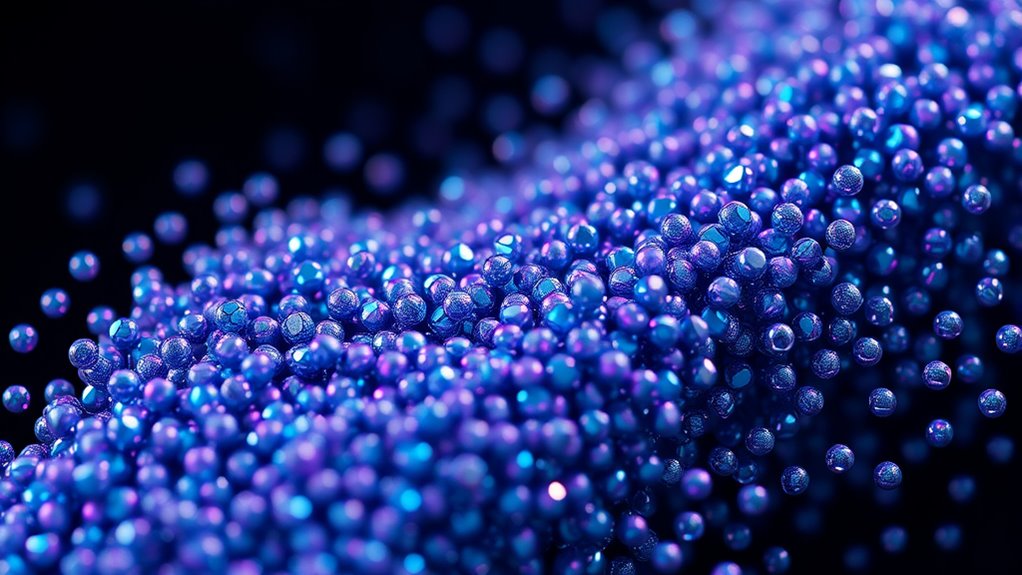
Building on the innovations in diagnostic nanotechnology, magnetic nanoparticles have emerged as powerful tools in cancer therapy. You can use iron oxide cores, valued for their biocompatibility and magnetic properties, to target tumors precisely. Surface coatings like polymers or silica prevent aggregation and improve compatibility, while attaching ligands or antibodies helps bind to specific cancer cell receptors. Using external magnetic fields, you guide these nanoparticles directly to tumors, reducing off-target effects. They can release drugs in response to pH changes in the tumor microenvironment or generate heat through magnetic hyperthermia, inducing cancer cell death. Some systems combine drug delivery with hyperthermia for enhanced effects. Additionally, these nanoparticles serve as MRI contrast agents, enabling real-time monitoring. Their multifunctionality offers promising advances in targeted, minimally invasive cancer treatments. The use of magnetic fields can also improve the accumulation of nanoparticles in tumor tissues, enhancing treatment efficacy. Moreover, understanding the fundamentals of magnetic resonance imaging can help optimize the design of these multifunctional systems for better clinical outcomes. Incorporating cybersecurity measures in the development and deployment of nanomedical devices can safeguard patient data and prevent malicious interference with treatment systems.
Sustainable Manufacturing in Nanochemistry
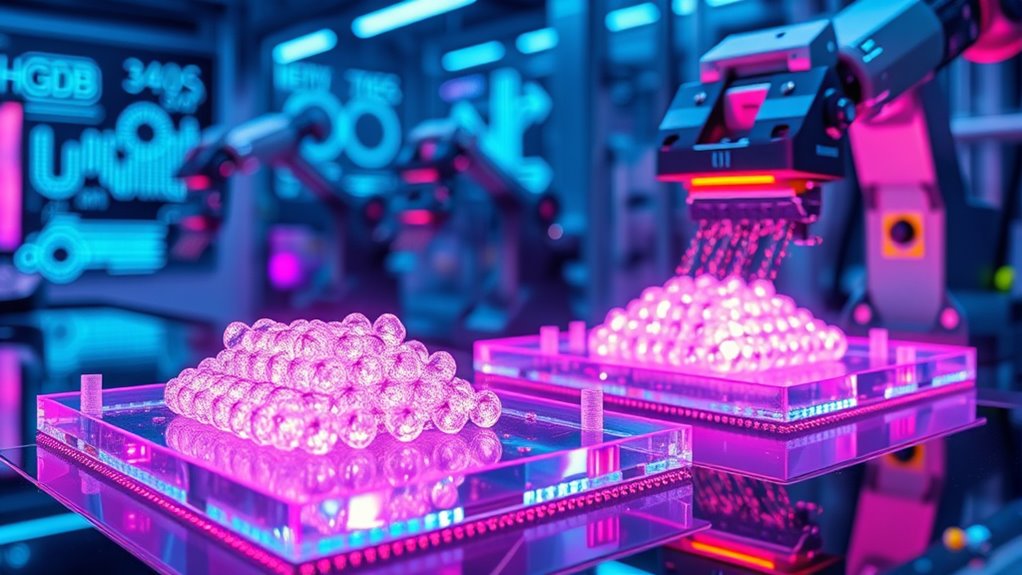
Sustainable manufacturing in nanochemistry is transforming how we produce advanced materials by emphasizing environmentally friendly practices and energy efficiency. You benefit from innovations like Berkeley Lab-developed nanosheets, which create recyclable barriers for electronics and packaging, reducing landfill waste. Techniques such as layer-by-layer nanopatterning enable precise control over material properties at industrial scales, while room-temperature synthesis minimizes energy use compared to traditional methods. Scalable self-assembly replaces complex lithography, streamlining nanoelectronics and energy storage production. Green synthesis methods like hydrothermal and microwave-assisted processes cut toxic waste and processing time, respectively. Addressing challenges such as nano-agglomeration through surface functionalization and continuous flow reactors ensures consistent quality. Additionally, implementing lifecycle analysis helps manufacturers evaluate environmental impacts throughout a product’s lifespan, promoting sustainable practices. With lifecycle analysis and energy recovery, manufacturers optimize resource use, aligning nanochemistry with sustainability goals.
Nanoencapsulation for Targeted Drug Delivery
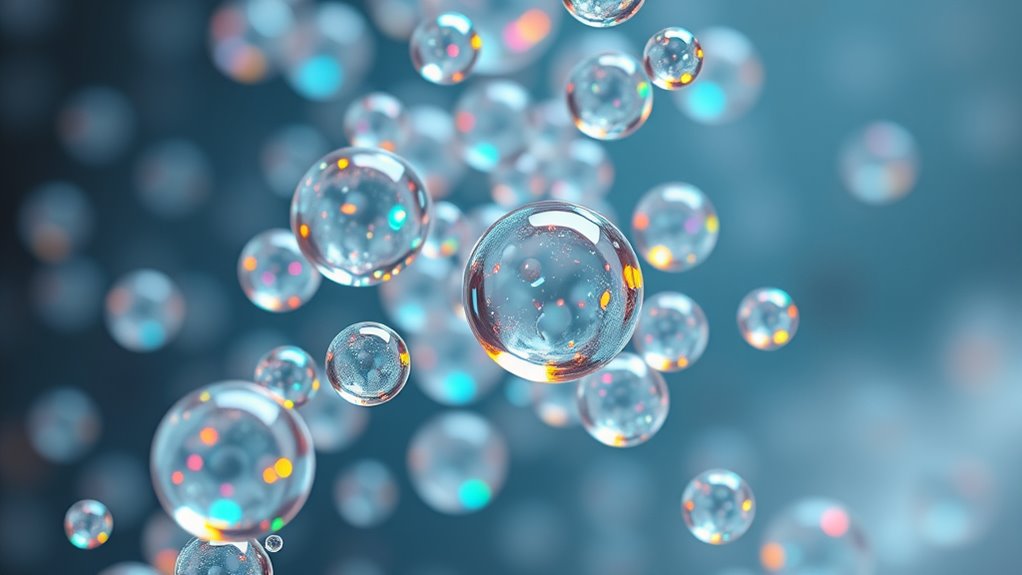
Nanoencapsulation technologies have revolutionized targeted drug delivery by enabling precise control over how and where medications are released in the body. You can now use polymeric nanoparticles, like PLGA or PLA, for controlled, biocompatible drug release. Liposomes and micelles efficiently encapsulate hydrophobic drugs, reducing systemic toxicity through passive targeting. Carbon nanotubes and dendrimers offer high drug-loading capacities and surface modifiability for active targeting. Magnetic nanocarriers allow external magnetic fields to guide delivery to specific tissues, minimizing off-target effects. Surface engineering, such as PEGylation, extends circulation time by evading immune detection. Receptor-specific targeting is achieved through antibody or ligand conjugation, while stimuli-responsive polymers release drugs in response to pH or temperature changes, ensuring precise, controlled therapy.
Atomic-Scale Manufacturing Techniques
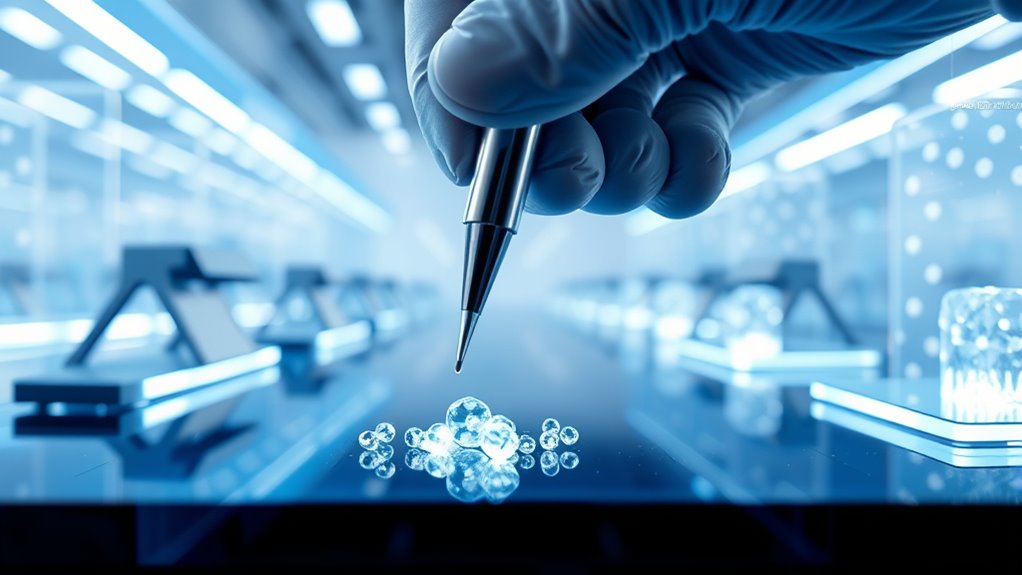
Advancements in nanochemistry have paved the way for atomic-scale manufacturing techniques that enable precise control over material structures at the atomic level. You can now synthesize materials with exact atomic arrangements using methods like Atomic Layer Deposition (ALD) and Atomic Layer Etching (ALE), though scalability and cost remain challenges. Techniques such as Scanning Tunnelling Microscopy (STM) and Atomic Force Microscopy (AFM) allow for direct imaging and patterning at the sub-nanometre scale, essential for creating nanoscale devices. Machine learning increasingly automates these processes, boosting efficiency and opening pathways for mass production. Despite technical hurdles, these tools are revolutionizing the development of highly specialized catalysts, quantum components, and energy materials, pushing nanochemistry toward practical, real-world applications.
Frequently Asked Questions
What Are the Environmental Impacts of Nanomaterials Disposal?
Did you know that about 95% of nanowaste ends up in wastewater treatment plants? You should realize that improper disposal of nanomaterials impacts ecosystems by contaminating soil, water, and air. These particles can bioaccumulate in marine life, disrupt plant growth, and alter microbial communities. Managing these risks is tough because detection, regulation, and long-term effects remain poorly understood, making disposal a significant environmental challenge.
How Does Nanochemistry Improve the Durability of Biomedical Implants?
Nanochemistry boosts the durability of biomedical implants by creating nanostructured surfaces and coatings that enhance mechanical strength and wear resistance. You benefit from improved material properties that withstand daily stresses and reduce degradation over time. Incorporating antimicrobial and bioactive nanoparticles also prevents infections and promotes better tissue integration. As a result, your implants last longer, perform better, and reduce the need for replacements, ensuring safer, more reliable medical solutions.
What Ethical Considerations Surround Nanotechnology Applications?
You should consider the ethical issues of nanotechnology applications, such as health and safety risks, environmental impact, and equitable access. You must guarantee that workers are protected from exposure, and that products are transparent about nanoparticle content. It’s also crucial to address privacy concerns, especially in medical uses, and advocate for fair distribution of benefits across different populations. Finally, you should support strong regulations to manage risks and promote responsible development.
How Are Nanomaterials Scaled up for Commercial Manufacturing?
Imagine building a skyscraper—your tools and methods determine success. To scale nanomaterials for manufacturing, you combine top-down carving for precision with bottom-up assembly for complexity. You use high-throughput techniques like aerosol processes and nanoimprint lithography to produce large quantities efficiently. You also monitor carefully to reduce defects and waste, ensuring safety and cost-effectiveness. This integrated approach turns tiny building blocks into massive industrial innovations.
What Are the Challenges in Integrating Nanochemistry With AI?
You face challenges integrating nanochemistry with AI because of complex, high-volume datasets that are hard to standardize and analyze in real-time. Your models often struggle with nanoscale behavior prediction and generalize poorly across different materials. Hardware limits, skill gaps, and high costs hinder adoption. Additionally, translating AI insights into reproducible experiments is tough, and regulatory or cybersecurity issues add further barriers to seamless integration.
Conclusion
You stand on the brink of a nanoscopic revolution, where each breakthrough acts like a star illuminating new paths in science and technology. As you delve deeper into these tiny marvels, you’ll access treasures hidden within atoms and molecules, transforming industries and lives. Embrace this frontier with curiosity and innovation, for in the domain of nanochemistry, even the smallest discovery can spark a universe of possibilities, shaping a future as intricate and boundless as the nanoscale itself.